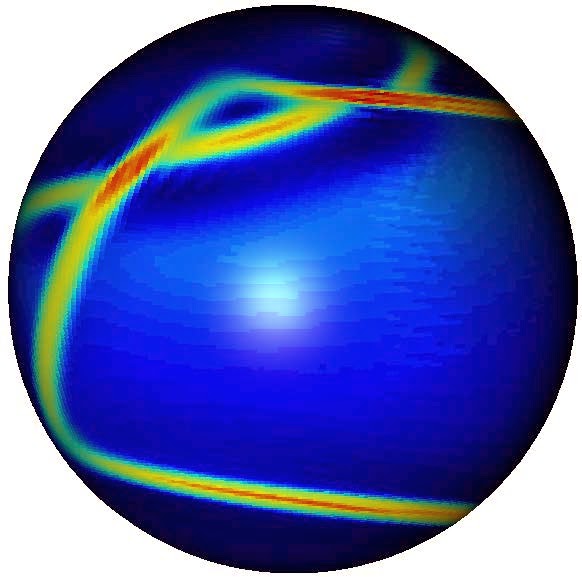
(Phys-org) —Two researchers, David Bercovici of Yale University and Yanick Ricard with the University of Lyon, have together used mathematical modeling to help explain how it was that our planet came to have tectonic plates and why they behave the way they do today. In their paper published in the journal Nature, the pair have explained how they performed mathematical analysis on observations of rocks today and combined data taken from prior theories to come up with a new model that might reflect Earth’s history during the first billion years of its existence.
The surface of planet Earth is covered in several moving plates which scientists believe is one of the major reasons that life was able to evolve and survive. But how the plates came to exist and wound up behaving the way they do today, has been mostly a mystery. In this new effort, the model built by the researchers offers one possible explanation.
It all comes down to grains of rock, they suggest—the smaller the grain, the weaker the rock. One type of rock, known as mylonite has been found to exist at every plate boundary on the planet, suggesting it has something to do with plate tectonics—when found, it is generally deformed with very small grains.
Bercovici and Ricard’s model starts with the idea that very early Earth was covered in hot mushy material. As that material began to cool, some parts or regions would cool faster or slower than others—the cooler parts would then naturally sink. Prior studies have shown that when such types of material sink, the surface becomes slightly deformed. With rock, scientists already known that deformations lead to weaker rock and subsequently smaller grains—and weaker rock would of course lead to additional deformations which suggests a feed-back loop would have evolved. On a planet-wide scale that would mean that weak zones would form at boundaries giving rise to the evolution of tectonic plates. Over billions of years, as the planet continued cooling, the result would be plate tectonics.
The same model can be used to explain why other planets in our solar system didn’t develop plates, and thus the conditions necessary for the development of life. Venus, for example, the numbers suggest, was simply too hot. If pre-plates developed, the deformations would heal due to the high temperatures, preventing the development of actual plates and possibly an atmosphere conducive to life.
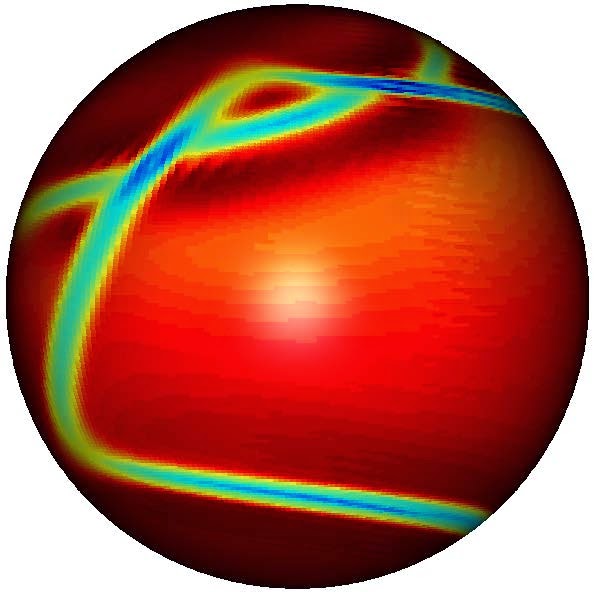
Abstract:
The initiation of plate tectonics on Earth is a critical event in our planet’s history. The time lag between the first proto-subduction (about 4 billion years ago) and global tectonics (approximately 3 billion years ago) suggests that plates and plate boundaries became widespread over a period of 1 billion years. The reason for this time lag is unknown but fundamental to understanding the origin of plate tectonics. Here we suggest that when sufficient lithospheric damage (which promotes shear localization and long-lived weak zones) combines with transient mantle flow and migrating proto-subduction, it leads to the accumulation of weak plate boundaries and eventually to fully formed tectonic plates driven by subduction alone. We simulate this process using a grain evolution and damage mechanism with a composite rheology (which is compatible with field and laboratory observations of polycrystalline rocks1, 2), coupled to an idealized model of pressure-driven lithospheric flow in which a low-pressure zone is equivalent to the suction of convective downwellings. In the simplest case, for Earth-like conditions, a few successive rotations of the driving pressure field yield relic damaged weak zones that are inherited by the lithospheric flow to form a nearly perfect plate, with passive spreading and strike-slip margins that persist and localize further, even though flow is driven only by subduction. But for hotter surface conditions, such as those on Venus, accumulation and inheritance of damage is negligible; hence only subduction zones survive and plate tectonics does not spread, which corresponds to observations. After plates have developed, continued changes in driving forces, combined with inherited damage and weak zones, promote increased tectonic complexity, such as oblique subduction, strike-slip boundaries that are subparallel to plate motion, and spalling of minor plates.
Video :
Note : The above story is based on materials provided by © 2014 Phys-org