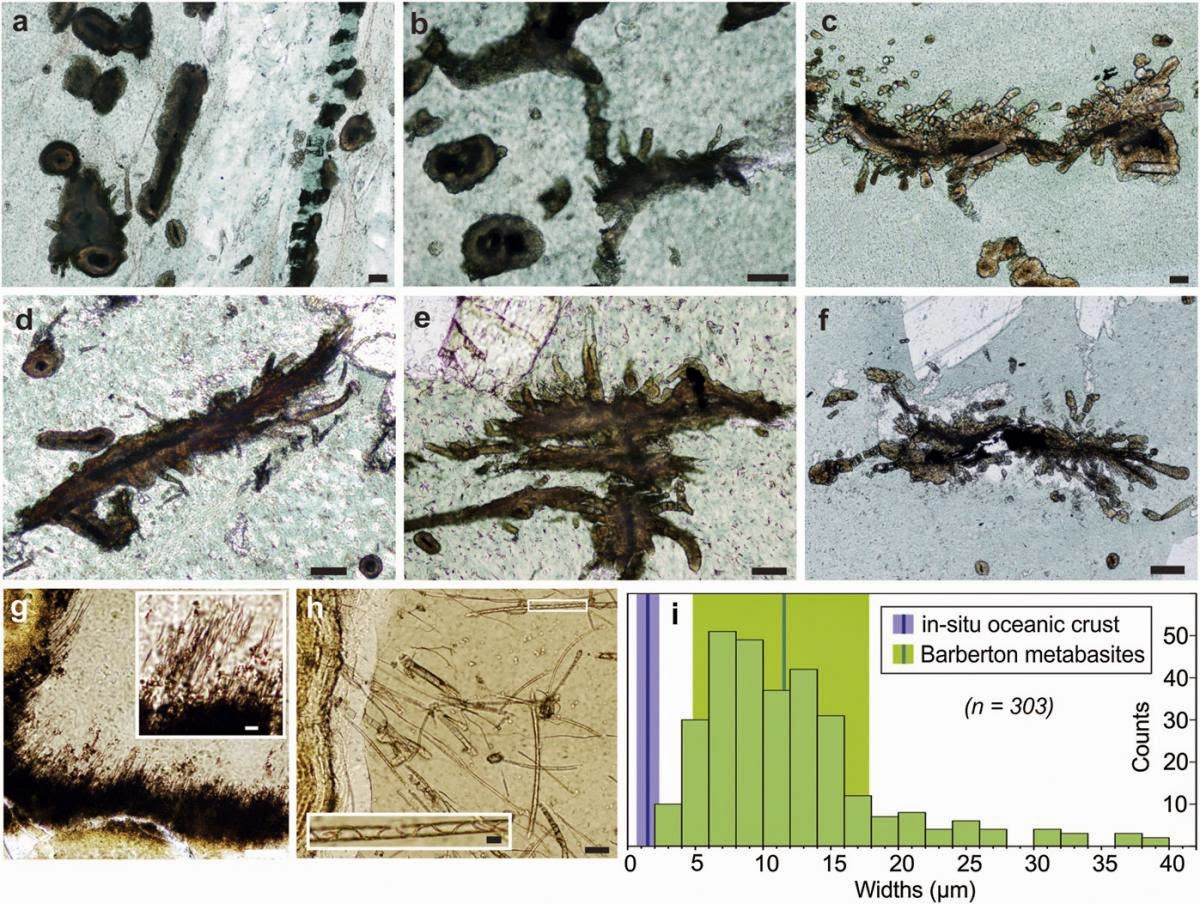
In the hunt for early life, geobiologists seek evidence of ancient microbes in the form of trace fossils – geological records of biological activity – embedded in lavas beneath the ocean floor. Filamentous titanite (a calcium titanium silicate mineral) microtextures found in 3.45 billion-year-old volcanic pillow lavas of the Barberton greenstone belt of South Africa, have been argued previously2 to be Earth’s oldest trace fossil, representing the mineralized remains of microbial tunnels in seafloor volcanic glass. However, scientists at the University of Bergen, Norway have reported new data based on in situ U-Pb (uranium-lead) dating, metamorphic temperature mapping constraints and morphological observations that bring the biological origin of these fossils into serious question.
The new age determined for the titanite microtextures is much younger than the eruptive and seafloor hydrothermal age of the previously proposed bioalteration model. As a result, the researchers have analyzed these fossils’ syngenicity (age as estimated by a textural, chemical, mineral, or biological feature formed at the same time as its encapsulating material) and biogenicity (any chemical and/or morphological signature preserved over a range of spatial scales in rocks, minerals, ice, or dust particles that are uniquely produced by past or present organisms). The scientists conclude that the oldest bona fide biogenic trace fossil now reverts to roughly 1.7 Ga microborings in silicified stromatolites found in China, and that the search for subsurface life – both on the early Earth as well as in extraterrestrial mafic–ultramafic rocks1, such as Martian basalts – be based not only on new biosignatures, but on new detection techniques as well.
Dr. Eugene G. Grosch discussed the paper that he and Dr. Nicola McLoughlin published in Proceedings of the National Academy of Sciences. “Previous work2 argued that titanite formed inside, or infilled, hollow tubes initially proposed to have been made by microbes living in the volcanic subseafloor at around 3.472 billion years ago – but the age estimate of the trace fossil formation and mineralization was not well constrained,” Grosch tells Phys.org. “In our PNAS study we took a critical approach, conducting a syngenicity test of the previous bioalteration model to determine if the titanite did indeed form 3.472 billion years ago during subseafloor hydrothermal alteration and Paleoarchean glass microbial bioalteration.” Using two laser-ablation inductively coupled plasma mass spectrometry, or LA-ICP-MS, instruments (single- and multi-collector), and a uranium-to-lead isotopic decay radiometric system, the scientists dated the titanite at roughly 2.9 billion years old – too recent for the titanite to be syngenetic with the 3.472 billion year old bioalteration model. “This was a challenge,” Grosch adds, “because these rocks are extremely old, so we had to be careful to take into account common lead in the titanite mineral.”
In order to potentially make a claim or confirm earliest candidate traces of life in Archean subseafloor environments, the researchers propose that careful geological work should first be conducted and that low-temperature metamorphic events should be completely characterized in Archean greenstone belt pillow lavas (bulbous, spherical, or tubular lobes of lava attributed to subaqueous extrusion). They accomplished this by using a new quantitative electron microprobe microscale mapping technique to map the composition of different minerals associated with the putative titanite filaments. In addition, they applied an inverse thermodynamic modelling approach to the mineral chlorite in the maps and calculated a metamorphic temperature map in the matrix surrounding a candidate titanite trace fossil. Their results showed, for the first time, constraints on metamorphic conditions and that on a microscopic scale, the best-developed titanite filaments were associated with the low-temperature microdomains. “This discovery indicates a cooling history around the titanite filaments, and supports an abiotic – that is, not associated with life – mineral growth mechanism at 2.9 Ga,” Grosch explains. “This proves that the titanite was a result of much younger metamorphic growth and not related to the posited biological activity in the 3.472 Ga bioalteration model constructed by previous investigators. Moreover, filamentous titanite cannot be used as a biosignature because it has failed a wide range of syngenicity and biogenicity tests.”
Finding that these titanite microtextures exhibit a morphological continuum bearing no similarity to candidate biotextures found in the modern oceanic crust also supports their conclusions. “One of the main lines of evidence in our study that questions the biogenicity of the titanite microtextures is the huge range of shapes and sizes that they exhibit,” Grosch tells Phys.org. “This contradicts the general principle accepted by palaeontologists that a fossil population should show a restricted size distribution that reflects biological control on growth, as opposed to self-organizing abiotic processes that do not show restricted size distributions. Furthermore, we argue that the growth continuum in the Barberton titanite microtextures, from oval-shaped hornfelsic (thermally metamorphosed rock) structures with few projections to coalesced oval-shaped structures that progress into bands with increasing number and size of filamentous projections, records an abiotic, metamorphic growth process and not the earlier seafloor trace fossil model. Lastly, in contrast to the microtextures of argued biogenic origin from the modern oceanic crust which do show a narrow size distribution and specific shapes, the Barberton microtextures show a much greater range in sizes – at least an order of magnitude greater – and a much larger spectrum of morphologies.”
To address the challenges encountered in their research, Grosch says that the key insight was that all the tests and in situ data indicated that titanite microtextures failed as a biosignature that represents Earth’s oldest trace fossil. “In addition,” he notes, “there are no organics such as decayed carbon or nitrogen associated with the titanite; the size, shape and distribution of the filamentous titanite are all not compatible with that expected for a biogenic population; the age is much too young at 2.8-2.9 billion years ago; and the quantitative petrological mapping indicates a thermal history compatible with an abiotic growth of titanite filaments, not as infilling minerals as previous studies have proposed.” (Petrology is the branch of geology that studies the origin, composition, distribution and structure of rocks.). Grosch concludes that filamentous titanite microtextures, such as those in the Barberton pillow lavas, can no longer be used as a biological search image for life in Archean metavolcanic glass, and that other search images combined with morphological and biogeochemical evidence for early life need to be found. “If we want to make a robust case for early life preserved in Archean volcanic rocks or any other ancient rock, we need to look for early morphological and biogeochemical biosignatures – but we also have to combine these with high-resolution 2- and 3-dimensional mapping and reconstructions,” he points out. “We also need to prove a ‘fossil’ is a very early structure preserved in the rock and not a later abiotic feature. We need to find new ways to carefully peel back layers of deep geological time and eliminate all abiotic scenarios first before we can be sure of an early body or trace fossil.”
Regarding biogeochemical traces of life on early Earth, the scientists have found that the sulfur isotopes of microscopic sulfide minerals found in the Barberton pillow lavas have unusually large fractionations (the ratio of light to heavy 32 to 34 sulfur atoms), and that this could record the activities of sulfur-based microbes in the Archean subseafloor. “In a previous study led by co-author Dr. Nicola McLoughlin3,” Grosch continues, “we suggested that these types of chemical signatures need to be further investigated as possible alternative evidence for an early subseafloor biosphere on early Earth. Such signatures are known from ancient sediments, where they are widely accepted as evidence of early sulfur based life forms – but this was the first and earliest evidence from subseafloor volcanic rocks.” In a previous work3, the scientists state that alternatives such as sulfur isotope fractionations recorded by basalt-hosted sulfides could be more promising in the search for evidence of ancient life. Grosch notes that today’s microbes use the light isotope in their metabolic pathways, such as 32S in microbial sulfate reduction. As a consequence, when seawater sulfate is used for energy by these microbes, the mineral pyrite, or FeS2, is formed as a reaction byproduct. As such, the fraction in the sulfur 32S/34 S ratio is large and can therefore be measured in the FeS2. “We can measure the pyrite 32S/34S ratio relative to an international standard derived from meteoritic sulfide and use the degree of fractionation as a biogeochemical marker. That’s a wide range of 32/34S ratios – and a negative range is a good geochemical sign of possible early Archean microbial life.”
Grosch also discusses the prospect of looking for signs of early life in extraterrestrial mafic-ultramafic rocks by adopting a highly critical and multi-pronged analytical testing approach towards biogenicity. “Until one day in the future when space missions return samples from Mars, we have to use satellite-based remote sensing techniques to investigate the abundant mafic-ultramafic rocks found on Mars.” (He adds that Martian meteorites are also of interest – particularly a group called Nakhalites that contain igneous minerals and are believed to show evidence of aqueous alteration and possible biosignatures) A good strategy,” he says, “would be to focus on locations where there’s strong evidence for water-rock interaction and preserved organic carbon, because these sites may have chemical gradients that could help sustain microbes.” In fact, in another study4 the scientists explore how microscale mapping of the low-temperature minerals in such rocks could be used to investigate their alteration history and to evaluate the possibility of preserving chemical and textural traces of life in extra-terrestrial mafic-ultramafic rocks.
“We need to look carefully for possible microbe morphologies and possible preserved microbial activity in extraterrestrial samples. We need to apply new thermodynamic and high-resolution analytical petrological techniques such as metamorphic, nano-SIMS and soft X-ray (synchrotron) mapping techniques to understand very low-temperature conditions of hydrothermal alteration and possible signs and preservation of microbial life in samples from other rocky planets, such as Mars.”
Moving forward, Grosch identifies the key next steps in their research and other possible innovations:
- Extensive geological mapping of the Barberton Greenstone Belt, South Africa to identify alternative locations and evidence for early microbial life
- Further studies of recent seafloor volcanic glass to establish if the microtunnels are really the product of microbial life – and if so, what type of microorganisms are involved
- Further geochronological work – that is, radiometric dating to better establish the timing of geological events and age of different environments in these ancient Archean rocks
- Development and refinement of thermodynamic models, in metamorphic petrology tools and in situ geochemistry techniques to better characterize and test microscopic textural and chemical evidence of putative life in Archean rocks
- Apply and compare multiple high-resolution techniques to candidate biosignatures in ancient rocks
Grosch notes that there are other areas of research that might benefit from their study. “In the field of paleontology, fossil experts now need to go and look for the oldest robust trace fossils – and while that our study questions the evidence in ancient metamorphic pillow lavas, and that the oldest bona fide candidate trace fossil comes from 1.7 billion year old rocks in China, if paleontologists look harder and in the right places, they may find trace fossils and evidence of microbial activities in older rocks, such as silicified seafloor sediments or in shallow marine Archean environments. In addition, from our findings we propose to astrobiologists and planetary scientists that looking for filamentous titanite microtextures as an extraterrestrial biosignature is misleading, and therefore they should seek other evidence for subsurface life on other wet rocky planets in our solar system – especially Mars – and possibly beyond.”
More information:
Reassessing the biogenicity of Earth’s oldest trace fossil with implications for biosignatures in the search for early life, Proceedings of the National Academy of Sciences, Published online before print on May 27, 2014, doi:10.1073/pnas.1402565111
Note : The above story is based on materials provided by © 2014 Phys.org