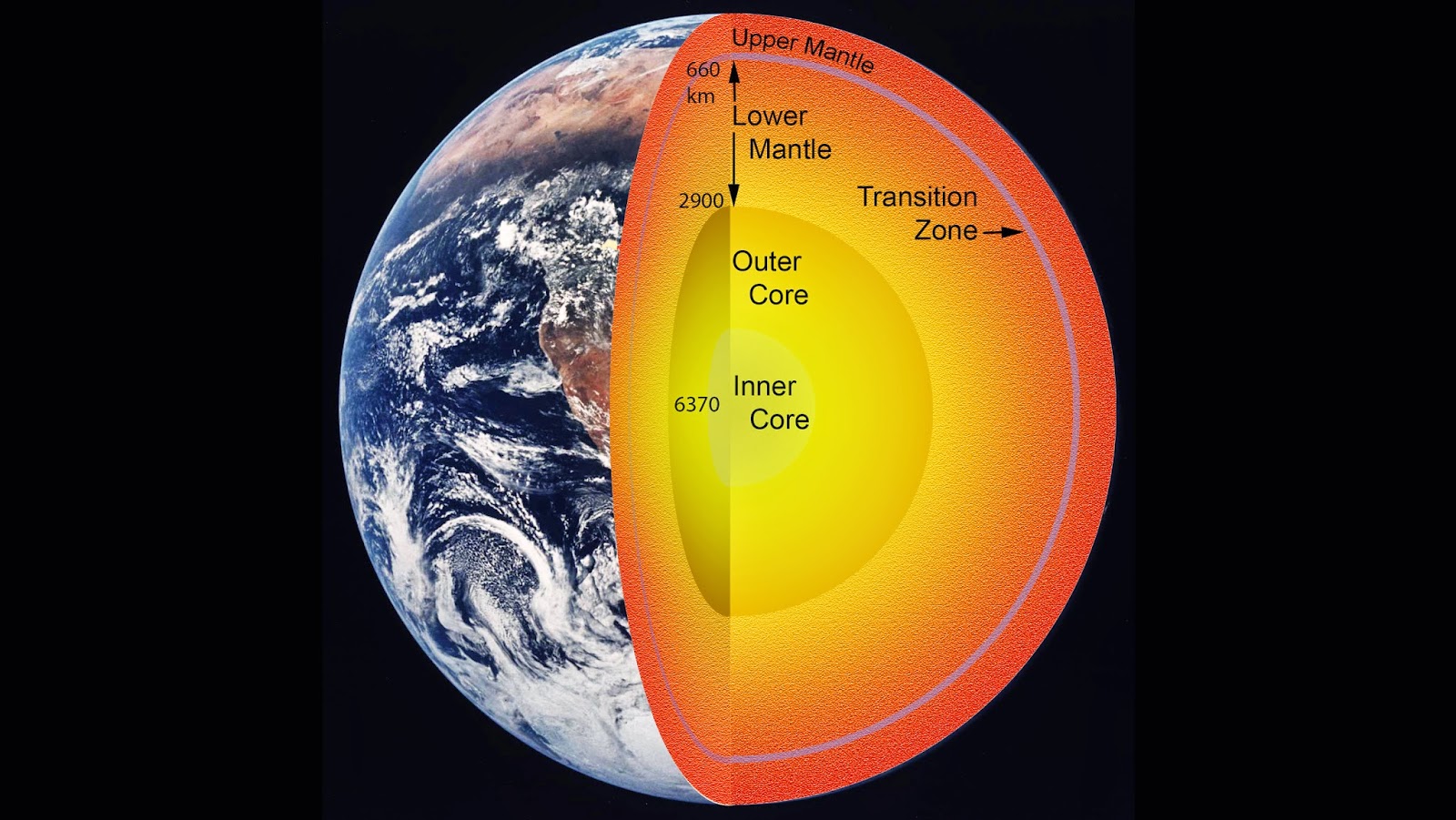
Researchers have found evidence of a potential “ocean’s worth” of water deep beneath the United States.
Although not present in a familiar form, the building blocks of water are bound up in rock located deep in the Earth’s mantle, and in quantities large enough to represent the largest water reservoir on the planet, according to the research.
For many years, scientists have attempted to establish exactly how much water may be cycling between the Earth’s surface and interior reservoirs through the action of plate tectonics. Northwestern University geophysicist Steve Jacobsen and University of New Mexico seismologist Brandon Schmandt have found deep pockets of magma around 400 miles beneath North America—a strong indicator of the presence of H₂O stored in the crystal structure of high-pressure minerals at these depths.
“The total H₂O content of the planet has long been among the most poorly constrained ‘geochemical parameters’ in Earth science. Our study has found evidence for widespread hydration of the mantle transition zone,” says Jacobsen.
For at least 20 years geologists have known from laboratory experiments that the Earth’s transition zone—a rocky layer of the Earth’s mantle located between the lower mantle and upper mantle, at depths between 250 and 410 miles—can, in theory, hold about 1 percent of its total weight as H₂O, bound up in minerals called wadsleyite and ringwoodite. However, as Schmandt explains, up until now it has been difficult to figure out whether that potential water reservoir is empty, as many have suggested, or not.
If there does turn out to be a substantial amount of H₂O in the transition zone, then recent laboratory experiments conducted by Jacobsen indicate there should be large quantities of what he calls “partial melt” in areas where mantle flows downward out of the zone. This water-rich silicate melt is molten rock that occurs at grain boundaries between solid mineral crystals and may account for about 1 percent of the volume of rocks.
“Melting occurs because hydrated rocks are carried from the transition zone, where the rocks can hold lots of H₂O, downward into the lower mantle, where the rocks cannot hold as much H₂O. Melting is the way to get rid of the H₂O that won’t fit in the crystal structure present in the lower mantle,” says Jacobsen.
He adds:
“When a rock starts to melt, whatever H₂O is bound in the rock will go into the melt right away. So the melt would have much higher H₂O concentration than the remaining solid. We’re not sure how it got there. Maybe it’s been stuck there since early in Earth’s history or maybe it’s constantly being recycled by plate tectonics.”
Seismic Waves
Melt strongly affects the speed of seismic waves—the acoustic-like waves of energy that travel through the Earth’s layers as a result of an earthquake or explosion. This is because stiff rocks, like the silicate-rich ones present in the mantle, propagate seismic waves very quickly. According to Schmandt, if just a little melt—even 1 percent or less—is added between the crystal grains of such a rock it causes it to become less stiff, meaning that elastic waves propagate more slowly.
“We were able to analyse seismic waves from earthquakes to look for melt in the mantle just beneath the transition zone,” says Schmandt.
“What we found beneath the U.S. is consistent with partial melt being present in areas of downward flow out of the transition zone. Without the presence of H₂O, it is very difficult to explain melting at these depths. This is a good hint that the transition zone H₂O reservoir is not empty, and even if it’s only partially filled that could correspond to about the same mass of H₂O as in Earth’s oceans,” he adds.
Jacobsen and Schmandt hope that their findings, published in the June issue of the journal Science, will help other scientists to understand how the Earth formed and what its current composition and inner workings are, as well as establish how much water is trapped in mantle rock.
“I think we are finally seeing evidence for a whole-Earth water cycle, which may help explain the vast amount of liquid water on the surface of our habitable planet. Scientists have been looking for this missing deep water for decades,” says Jacobsen
Mantle Rock Studies
The study combined Schmandt’s analysis of seismic data from the USArray, a network of over 2,000 seismometers across the U.S., with Jacobsen’s laboratory experiments, in which he examined the behaviour of mantle rock under conditions designed to simulate the high pressures and temperatures present at 400 miles below the Earth’s surface.
The USArray is part of Earthscope, a program sponsored by National Science Foundation. Jacobsen’s experiments were conducted at two Department of Energy user facilities, the Advanced Photon Source of Argonne National Laboratory and the National Synchrotron Light Source at Brookhaven National Laboratory.
Taken as a whole, their findings produced strong evidence that melting may occur about 400 miles deep in the Earth, with H₂O stored in mantle rocks, such as those containing the mineral ringwoodite, which is likely to be a dominant mineral at those depths.
Schmandt explains that he made this discovery after carrying out seismic imaging of the boundary between the transition zone and lower mantle. He found evidence that, in areas where “sharp transitions” like melt are present, some earthquake energy had converted from a compressional, or longitudinal wave, to a shear or S-wave. The phase of the converted S-waves in areas where the mantle is flowing down and out of the transition zone indicated a significantly lower velocity than surrounding mantle. The discovery suggests that water from the Earth’s surface can be driven to such great depths by plate tectonics, eventually resulting in the partial melting of the rocks found deep in the mantle.
“We used many seismic wave conversions to see that many areas beneath the U.S. may have some melt just beneath the transition zone. The next step was comparing these areas to the areas where mantle flow models predict downward flow out of the transition zone,” says Schmandt.
Ringwoodite
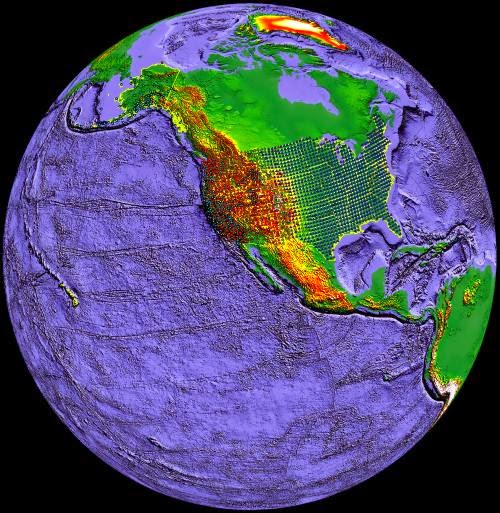
Schmandt and Jacobsen’s findings build on a discovery reported in March in the journal Nature in which scientists discovered a piece of the blue mineral ringwoodite inside a diamond brought up from a depth of 400 miles by a volcano in Brazil. That tiny piece of ringwoodite—the only sample we have from within the Earth—contained a surprising amount of water bound in solid form in the mineral.
“Not only was this the first terrestrial ringwoodite ever seen—all other natural ringwoodite examples came from shocked meteorites—but the tiny inclusion of ringwoodite was also full of H₂O, to about 1.5 percent of total weight,” says Jacobsen. “This is about the maximum amount of water that we are able to put into ringwoodite in laboratory experiments.”
Although the discovery provided direct evidence of water in the deep mantle at about 700 kilometers (434 miles) deep, the diamond sampled only one point of the mantle. Jacobsen explains that the paper expands the search to question how widespread hydration might be throughout the entire transition zone. This is important because the presence of H₂O in the large volumes of rock found at depths of between 410 to 660 kilometers (255 to 410 miles) would “significantly alter our understanding of the composition of the Earth.”
“It would double or triple the known amount of H₂O in the bulk Earth. Just 1 to 2 percent H₂O by weight in the transition zone would be equivalent to 2 to 3 times the amount of H₂O in the oceans,” adds Jacobsen.
Big Questions
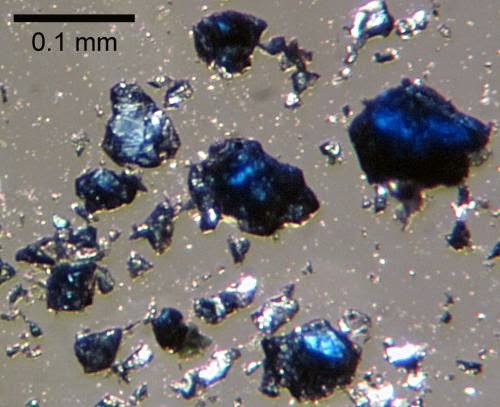
Looking ahead, Jacobsen admits that some big questions remain. For example, if the transition zone is full of H₂O, what does this tell us about the origin of Earth’s water? And is the presence of ringwoodite in a planet’s mantle necessary for a planet to retain enough original water to form oceans? Moreover, how is the H₂O in the transition zone connected to the surface reservoirs? Is the transition zone, if it contains a geochemical reservoir of H₂O larger than the oceans, somehow buffering the amount of liquid water on the Earth’s surface?
“An analogy could be that of a sponge, which needs to be filled before liquid water can be supported on top. Was water in the transition zone added through plate tectonics early in Earth’s history, or did the oceans de-gas from the mantle until an equilibrium was reached between surface and interior reservoirs?” asks Jacobsen.
Either way, the research is likely to be of strong interest to astrobiologists largely because water is often so closely linked to the formation of biological life. Remote geochemical analysis could be one way of detecting if such processes occur elsewhere in the universe, and it is likely that such analysis would involve the use of gamma-ray, neutron, and x-ray spectrometers of the type used by the NASA MESSENGER spacecraft for the remote geochemical mapping of Mercury.
“On other hard to reach planets it’s not practical to apply the type of seismic imaging that I used. So my guess is that geochemical analysis of volcanic rocks from other planetary bodies may be our best way to test whether volatiles are stored in the planet’s interior,” says Schmandt.
Note : The above story is based on materials provided by Astrobio.net
This story is republished courtesy of NASA’s Astrobiology Magazine.