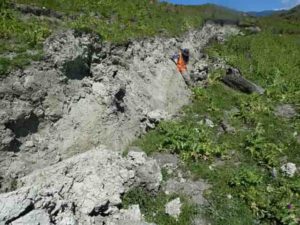
Slip-parallel grooves (striations) on fault surfaces are considered a robust indicator of fault slip direction, yet their potential for recording aspects of earthquake rupture dynamics has received little attention. During the 2016 Kaikōura earthquake (South Island, New Zealand), >10 m of dextral strike-slip on the steeply dipping Kekerengu fault exhumed >200 m2 of fresh fault exposure (free faces) where it crossed bedrock canyons. Inscribed upon these surfaces, we observed individual striae up to 6 m long, all of which had formed during the earthquake. These were typically curved. Using simulations of spontaneous dynamic rupture on a vertical strike slip fault, we reproduce the curved morphology of striae on the Kekerengu fault.
Assuming strike-slip pre-stress, our models demonstrate that vertical tractions induced by slip in the so-called cohesive zone result in transient changes in slip direction. We show that slip-path convexity is sensitive to the direction of rupture propagation. To match the convexity of striae formed in 2016 requires the rupture to have propagated in a northeast direction, a prediction that matches the known rupture direction of the Kaikōura earthquake. Our study highlights the potential for fault striae to record aspects of rupture dynamics, including the rupture direction of paleo strike-slip earthquakes.
The direction of earthquake rupture propagation exerts a profound influence on the distribution of strong ground shaking. As a consequence, damage and loss experienced by a community during an earthquake is dependent on the community’s location relative to the direction of rupture propagation—a relationship especially true for large strike-slip events (Somerville et al., 1997; Gomberg et al., 2001).
This effect has been observed in many large magnitude strike-slip earthquakes in recent decades; e.g., the 1992 Mw 7.3 Landers (California) earthquake (Velasco et al., 1994), the 2002 Mw 7.9 Denali (Alaska) earthquake (Eberhart-Phillips et al., 2003), and the 2018 Mw 7.5 Palu (Indonesia) earthquake (Bao et al., 2019). Based on historical data, it is uncertain whether large magnitude earthquakes systematically repeat their rupture direction; moreover, establishing the direction of past earthquakes using geological data has proved difficult. Previous studies have used structural data such as branching fault intersections (Poliakov et al., 2002; Fliss et al., 2005) and off-fault damage patterns in both ancient fault zones (Di Toro et al., 2005) and recent surface ruptures (Klinger et al., 2018) to infer rupture direction. However, these studies lack a context of seismological data with which to verify the rupture direction during a particular event.
Based on our literature review, out of ~55 surface ruptures observed geologically in the field since the late 1800s (Wesnousky, 2008; Biasi et al., 2013), 20 of the field datasets include measurements of coseismic slickenlines. Of these, 70% are curved, indicating that coseismic changes in slip direction are common (see the GSA Data Repository1 and references therein). Spudich et al. (1998) used the curvature of slickenlines (up to 50°) found on the Nojima fault following the 1995 Kobe (Japan) earthquake to infer the magnitude of shear traction on the fault surface. However, aside from this pioneering work, the potential link between curved slickenlines and the dynamics of earthquake rupture has received little attention. In this study, we propose that striae produced on fault plane surfaces during coseismic slip can reveal aspects of the dynamic rupture process.
We present new slickenline data from fault surfaces that were exposed during the 2016 Mw 7.8 Kaikōura earthquake (South Island, New Zealand)—an event that has a well-established southwest-northeast rupture direction across ~200 km of the Pacific-Australian plate boundary in New Zealand (Hamling et al., 2017; Kaiser et al., 2017; Ando and Kaneko, 2018; Litchfield et al., 2018) (Fig. 1A). We observed curved coseismic striae on multiple fault outcrops along a 10 km section of the Kekerengu fault, the structure that yielded the greatest slip and moment release during this earthquake (Cesca et al., 2017; Holden et al., 2017). The systematic convexity of these striae records temporal changes in the coseismic slip direction on this fault. To uncover the mechanism that was responsible for the observed variation in slip direction, we undertook dynamic rupture modeling of a vertical strike-slip fault, using a simple set of boundary conditions. Here we discuss the potential for curved striae to record the rupture direction of paleo strike-slip earthquakes.
Curved Striae on the Kekerengu Fault
The Kekerengu fault is part of the Marlborough Fault System, a series of mainly dextral faults that transfer slip between the Hikurangi megathrust and the Alpine fault. Dextral offset of Pleistocene terraces and uplift of the Seaward Kaikōura Ranges on its western side show that the Kekerengu fault is primarily strike-slip, with a lesser reverse component (Van Dissen et al., 2016). During the Kaikōura earthquake, many stream canyons were dextrally displaced 8–10 m and uplifted on their northwest sides by 1–2 m across the Kekerengu fault, which dips steeply to the northwest (>65° dip) (Kearse et al., 2018). Offset of these steep canyon walls (slopes of 20–30°) exhumed more than a dozen smooth and near-planar fault surfaces that were ≤7 m long in the slip direction, each coated in a 1–2 cm layer of soft fault gouge. Nine of these free faces hosted coseismic fault striae that were curved. For example, Figure 1D depicts a striation where slip initiated as dextral-reverse motion, and as further displacement accrued, the direction of slip curved to become nearly pure strike-slip.
This convex-up slip path was repeated at six out of the nine sites that contained curved striae, with some sites containing many measurement locations where striae were recorded. Curved striae were found mostly on the upper part of the free face, suggesting they were formed early in the rupture. The angular magnitude of the observed convexity is typically ~15° and is >40° at sites 3 and 7. At two sharp bends in the fault trace, the sense of uplift was opposite to the dominant one, and their striae describe a convex-down slip path. In all cases, the incremental magnitude of fault slip—both vertical and dextral—recorded by the individual fault striae segments (0.2–6 m) amounts to only a fraction of the known coseismic net displacement at these sites, which was 8–10 m.
Post-earthquake erosion of free faces in the days to months following the earthquake undoubtedly curtailed the length of individual straie; however, some striae become increasingly diffuse toward their ‘downstream’ ends , suggesting a different process is responsible for their limited length. Near-fault geodetic and seismological data collected at two stations (the KEKS seismic station, and a high-rate continuous GPS station located 30 km northeast of the study area) on the northwest side of the Kekerengu fault during the earthquake (Fig. 2A; see the Data Repository) imply that the initial ~5 s of rupturing on the Kekerengu fault accumulated up to 4 m of almost pure dextral-slip and <0.3 m of throw, whereas most of the throw (1–2 m) began accumulating 4–7 s after this. The available geological and geophysical data suggest that the curved striae on the Kekerengu fault were inscribed during the early phase of strike-slip–dominated rupturing.
Dynamic Rupture Model
To evaluate possible mechanisms for the curvature of Kekerengu fault striae, we simulated the spontaneous, dynamic rupture of a 50-km-long and 25-km-deep vertical strike-slip fault in layered elastic half space, using a spectral-element code (Ampuero, 2002; Kaneko et al., 2008). A horizontal initial shear traction was applied to the fault surface, with a magnitude that increased linearly with depth. Dynamic rupture was initiated by overstressing a small 3-km-wide patch in the center of the fault at a depth of 12 km, and then the rupture propagated spontaneously across the fault surface, where it was governed by slip-weakening friction laws (for additional details of the model setup, see the Data Repository).
Slip paths for 42 nodes on the fault surface (gray arrows in Fig. 3C) depict cseismic motion of the near-side of the fault relative to the far side. Their lengths depict slip magnitudes, and their shape and curvature illustrates any changes in slip direction. At depths >3 km, slip paths are horizontal and show little change in slip direction with time. For nodes located along mixed-mode rupture directions (Andrews, 1994) at depths <3 km, slip paths are more complex. At depths <3 km, slip follows a curved path during the initial few decimeters; at locations between x = 0 and x = –20 km, curvature is convex-up (Fig. 3A), while on the other side of the hypocenter between x = 0 and x = 20 km (right side of Fig. 3C), curvature is convex-down. As further displacement is accrued, slip directions converge toward a horizontal rake of 180°. These results do not necessarily imply that one side of the fault experiences an opposing sense of net uplift at either end of a rupture (this is only true for cases with pure strike-slip pre-stress). Rather, our results suggest that the convexity of the initial slip path is opposite at either end of the rupture, regardless of the rake of initial shear traction on the fault.
Mechanism for Changes in Slip Direction
The mechanism behind transient changes in slip direction in our dynamic model is consistent with the one proposed by Spudich et al. (1998). Dynamic changes in rake angle are driven by vertical stress changes along mixed-mode rupture directions produced within the so-called cohesive zone (the part of the fault that is slipping but has not yet fully weakened). Seismic waves are continuously generated within this zone, and they carry vertical stresses that induce vertical components of slip on the sliding surface.
At depths below 3 km, confining stresses are large compared to the dynamic loads, a relationship that suppresses the magnitude of these transient vertical motions. Yet slip at these depths retains subtle (<2°) temporal changes in slip direction, and exhibits the same reversal in path convexity on either side of the hypocenter (left versus right sides of Figure 3C are yellow and green, respectively; see the Data Repository).
Near Earth’s surface, where confining stresses are low, the ratio of dynamic to confining stress is larger, promoting a larger magnitude of coseismic vertical slip. As the rupture front propagates along strike, the model predicts opposite senses of slip deflection near the two propagating rupture tips: convex-upward on one side and convex-downward on the other. Figure 4 details changes in shear traction and slip velocity at the rupture tip to highlight the correlation between vertical stress and rotation of the slip direction.
Discussion and Conclusions
We note a correlation between the convexity of curved striae on the Kekerengu fault and results of our dynamic rupture model. As slip initiates, movement of the downstream side of the fault (the side moving in the direction of rupture propagation) follows a convex-up slip path that initially has a rake of ~15° to (and ultimately becomes parallel with) the direction of initial shear stress on the fault (Fig. 3A). Although several of our sites—specifically those at sharp fault bends—showed the opposite sense of slip-path convexity, we suggest that the kinematic differences at these areas induced local stresses that overwhelmed the more general dynamic effects described by our model. We therefore interpret the predominant pattern of slip-path curvature on the Kekerengu fault to have resulted from vertical stress changes in the cohesive zone of the northeast-propagating rupture of the Kaikōura earthquake. In addition, the curvature of striae seen on multiple exposures of the Nojima fault following the 1995 Mw 7.2 Kobe (Japan) earthquake (Otsuki et al., 1997) is consistent with our model, and with the known direction of rupture propagation in this event (Yoshida et al., 1996). These dynamic effects are likely not to be restricted to strike-slip faults, as mixed-mode dynamic rupture on dip-slip faults should also produce transient changes in initial slip direction, which may explain curved striae seen after events such as the 1954 M 7.0 Fairview Peak (Nevada, USA) (Slemmons, 1957) and 2011 Mw 6.6 Fukushima (Japan) earthquakes (Otsubo et al., 2013).
We offer two possible explanations for why striae on the Kekerengu fault capture only an early and small (typically <10% of total) increment of the 2016 slip. One possibility is that, at the onset of slip, the fault plane (in the near surface) was rough compared to the final stages of slip, having only just broken any cohesion gained during the interseismic period. If so, this might promote asperity ploughing and striae production at that early stage (Tesei et al., 2017), thus promoting a temporal bias in the striation record. According to this scenario, the early-formed asperities (i.e., topography in the new fracture surface) were progressively abraded during the accumulating slip, resulting in a smoother fault plane and a termination in striation production. Another possibility (and one that is not mutually exclusive with the former) is that during slip in the near-surface, along-strike translation of large (meter-scale) corrugations in the fault surface (e.g., Candela et al., 2012) promoted transient separation of the fault walls, thereby terminating the engraving process. Both ideas are supported by individual striae that are deeply inset near their inception point but are shallower and more diffuse toward their termination.
Spudich et al. (1998) together with Guatteri and Spudich (1998)—both of which assume depth-independent pre stress—suggest that the fractional stress drop, defined as the ratio of the stress drop to the magnitude of initial shear stress, is ~0.7 on faults that exhibit large (>30°) changes in coseismic slip direction. In our model that features depth-dependent stress, in order to reproduce up to ~20° changes in slip direction on the Kekerengu fault, the fractional stress drop is ~0.2, which corresponds to the ‘high stress’ case of Guatteri and Spudich (1998). Even in the case of ‘low stress’, the pattern of slip-path convexity should still depend on rupture direction.
Striated fault surfaces are routinely measured in neotectonic and paleoseismic studies, including on well preserved Holocene scarps and on excavated fault surfaces in paleoseismic trenches. Crosscutting or overlapping striae seen in such places do not necessarily represent the superposition of multiple earthquakes; and our results provide a simple explanation for transient changes in slip direction during a single slip episode, which can create overlapping striae (e.g., the 2011 Fukushima earthquake; Otsubo et al., 2013). More importantly, our study demonstrates that it may be possible to infer the rupture direction of paleoearthquakes in cases where coseismic striae record temporal changes in slip direction. To do this, we propose that data be collected from structurally simple parts of the fault that are representative of its overall kinematics, and that are also likely to generate and preserve striae. We further suggest that multiple sites be evaluated to establish any systematic patterns in striation convexity.
Temporal changes in slip direction during dynamic rupture are likely to be a widespread characteristic in the near-surface during large-magnitude earthquakes. Geological evidence of this type, when combined with simulations of dynamic rupture, may yield important insight into the near-field dynamics of fault slip, including the rupture direction of paleo strike-slip earthquakes and, by inference, the rupture direction of future strike-slip earthquakes; thereby helping to better constrain the distribution of damaging ground shaking in those events.
Reference:
Jesse Kearse, Yoshihiro Kaneko, Tim Little, Russ Van Dissen; Curved slickenlines preserve direction of rupture propagation. Geology doi: https://doi.org/10.1130/G46563.1
Note: The above post is reprinted from materials provided by Geological Society of America.