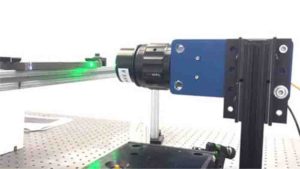
It seems like a smooth slab of stainless steel, but look a little closer, and you’ll see a simplified cross-section of the Los Angeles sedimentary basin.
Caltech researcher Sunyoung Park and her colleagues are printing 3D models like the metal Los Angeles proxy to provide a novel platform for seismic experiments. By printing a model that replicates a basin’s edge or the rise and fall of a topographic feature and directing laser light at it, Park can simulate and record how seismic waves might pass through the real Earth.
In her presentation at the Seismological Society of America (SSA)’s 2021 Annual Meeting, Park explained why these physical models can address some of the drawbacks of numerical modeling of ground motion in some cases.
Small-scale, complex structures in a landscape can amplify and alter ground motion after an earthquake, but seismologists have a difficult time modeling these impacts, said Park. “Even though we know that these things are very important to ground shaking, the effects of topography, interfaces and edges are hard problems to study numerically.”
Incorporating these features in ground motion simulations requires a lot of computational power, and it can be hard to verify these numerical calculations, she added.
To address these challenges, Park began creating 3D models of simple topographical and basin features to explore these effects on ground shaking. Metal is her preferred printing material, “because it can be as rigid as the conditions at the Earth’s lower crust,” she said.
By controlling the printing parameters, Park can also control the density of the metal as it is laid down by the printer, creating a material with different seismic velocities. The result, in the case of the Los Angeles basin example that she showed at the meeting, is a 20 by 4-centimeter model that represents a 50-kilometer cross-section through the basin.
At a scale of about 1:250,000 for the printed landscape, Park needed to scale down the wavelengths that she used to simulate seismic waves as well, which is where the laser-based source and receiver system comes in. A laser shot at the model mimics a seismic source event, and laser doppler receivers sense the resulting vibrations as the seismic waves interact with the model’s features.
Experiments with the models have yielded some intriguing findings. With a shallow basin cross-section, for instance, Park found that some of the high-frequency waves were blocked from traveling across the basin.
“We know that basins are usually amplifying ground motions,” she said, “but this suggests we should be thinking about that in terms of different frequency contents as well.”
Park said the models might also be useful for studying wave propagation through other seismologically complex features, such as highly damaged rock near a fault, rock layers injected with fluids and gases during oil and gas extraction or carbon sequestration, and features in the deep Earth.
Park will join the Department of Geophysical Sciences at The University of Chicago in June 2021.
Note: The above post is reprinted from materials provided by Seismological Society of America.