Fluorite
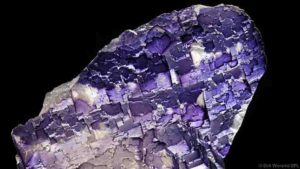
Fluorite can appear to be almost any colour (Credit: Dirk Wiersma/SPL)
Forget rubies, garnets and sapphires. Fluorite may be the world’s most colourful mineral, because of the enormous range of brilliant and even iridescent colours it displays.
The funny thing is, pure fluorite crystals are transparent.
A crystal’s colour is dictated by the way light interacts with the chemicals in it, and by how these are bonded in an orderly structure, or lattice. Any impurities that work their way into fluorite’s lattice can alter its apparent colour. For example, manganese ions turn it orange.
Structural defects within the lattice, known as colour centres, have a similar effect.
Fluorite’s hallmark deep purple hue is the result of a small number of fluoride ions being permanently forced out of their lattice positions by irradiation or heating. When they move, an electron is left behind in each hole. When light hits the crystal, it is absorbed and re-emitted by these electrons, producing the colour we see.
Some fluorite specimens even have bands of different colours.
Fluorite forms in hydrothermal veins in the Earth’s crust and in cavities in sedimentary rocks. Over the centuries, these fissures are constantly opening and closing, sometimes cutting off the fluids needed for fluorite to form. It’s the subtle changes in the chemistry of these fluids that causes colour zoning in the crystals as they grow.
Selenite
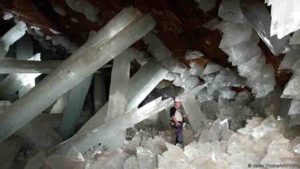
Giant selenite crystals in the Cueva de los Cristales (Credit: Javier Trueba/MSF/NPL)
Buried beneath the Sierra de Naica mountain in Chihuahua, northern Mexico, the Cueva de los Cristales (Cave of Crystals) is home to the largest crystals on planet Earth.
Gargantuan, milky white beams of selenite, some as long as 11m and more than 1m wide, criss-cross the underground chamber. “There is no other place on the planet where the mineral world reveals itself in such beauty,” says Juan Manuel García-Ruiz of the University of Granada in Spain, a geologist who studies the crystals.
The crystals were discovered in 2000 by two brothers excavating new tunnels in the Naica mine, in search of fresh reserves of zinc, silver, and lead.
The cavity, which measures about 10m by 30m, had previously been flooded with heated water. Only when the miners started pumping it out were the monumental structures revealed.
In 2007, García-Ruiz and his team figured out how the crystals were able to grow so big.
Around 26 million years ago, volcanic activity beneath the mine filled the cave with hot water rich in the mineral anhydrite. Anhydrite is stable above 58 °C, but as the underlying magma cooled, it dissolved into the surrounding water.
Very slowly, over hundreds of thousands of years, its chemical components reassembled as gypsum, which can take the form of crystals. Large elongate crystals of gypsum are known as selenite.
Within the Cueva de los Cristales, the temperature has consistently hovered around the magic 58 °C mark ever since.
Another crystal cave, discovered closer to the surface in Naica, also contains selenites. They are still spectacular at about 1m in length, but not as large as those of the Cueva de los Cristales, because this cave cooled faster.
Iceland Spar
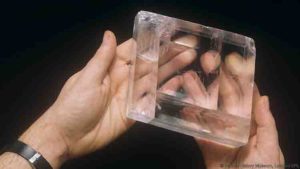
The Icelandic sagas of the 10th century record the details of Viking voyages. They describe a mysterious “sunstone”, which Scandinavian seafarers used to locate the Sun in the sky and navigate on cloudy days.
The identity of the stone stumped scholars for centuries, but in 2011 a convincing candidate was put forward: Iceland spar.
This clear variety of calcite is common in Nordic regions. It bends light by two different amounts, producing a double image (see the picture above).
This property is called birefringence. It’s caused by discrepancies in the binding forces that hold the atoms of the crystal together. The forces are stronger in some directions than others.
When light passes through calcite crystals, it is split into two rays. The asymmetry in the crystal’s structure causes the paths of these two beams to be bent by different amounts, resulting in a double image.
How did that help the Vikings? Researchers studied a piece of Iceland spar discovered aboard an Elizabethan ship that sunk in 1592. They found that moving the stone in and out of a person’s field of vision causes them to see a distinctive double dot pattern that lines up with the direction of the hidden Sun.
Quartz
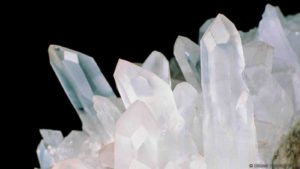
Quartz also does interesting things because of its structural asymmetries.
If you squeeze a crystal of quartz, it generates a tiny electric current. The pressure on the crystal’s surface forces ions within it to move out of position, upsetting the overall charge balance and turning the crystal into a tiny battery, with oppositely-charged faces.
The phenomenon is known as the piezoelectric effect, and it also works in reverse. Pass an electric current through a quartz crystal, and it will squeeze itself.
Quartz watches use tiny slivers of cut quartz as oscillators to keep precise time. Electricity from the watch battery causes the crystal to oscillate thousands of times per second, and circuits in the watch convert these oscillations to a once-per-second digital beat.
Quartz was also central to our developing understanding of crystals. In 1669, Danish scientist Nicolas Steno noticed that quartz crystals, irrespective of where on Earth they were found, always showed the same angles between similar crystal faces.
By the turn of the 19th century, French crystallographer René Just Haüy had extended this idea. He realised that the same rules underlie the shapes and angles of all crystals.
We now understand that the shapes of crystals are an expression, on a grand scale, of the orderly lattices in which their constituent atoms are arranged.
Galena
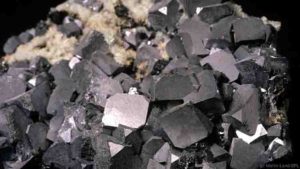
Galena is the most common lead-rich mineral, and an important ore of both lead and silver. But that’s just its day job.
It’s the crystal’s ability to extract music and voices from radio waves that makes it truly beguiling. It put galena centre stage in the revolutionary crystal radio sets of the early 1900s.
Galena is a semiconductor, meaning that it will conduct electricity under certain circumstances. In metals, free electrons flow as electricity when a voltage is applied. In galena – a non-metal – small crystal impurities or imbalances in its chemical proportions create a situation where, if electrons can be excited enough, they can be ripped from their atoms and made to flow.
In a crystal radio set, a fine metal wire known as a “cat’s whisker” rests delicately on the surface of a galena crystal. This combination allows current to pass easily in one direction but not the other. This converts the oscillating radio waves picked up by an antenna into an electric signal that can be transformed into sound by speakers.
Not every position on the crystal will perform, so fiddling with the cat’s whisker to find a sweet spot takes patience and skill.
Extra-terrestrial carbon crystals
Diamond is the hardest known natural material on Earth. It is the industry standard for grinding, cutting, drilling and polishing jobs.
But two new kinds of ultra-hard carbon crystals, found embedded in a Finnish meteorite in 2010, put the precious stone to shame.
The Haverö meteorite crashed to Earth in 1971. When researchers used diamond paste to polish a slice, they noticed something extraordinary: small pockets of material emerging in relief from the surface. When they analysed the stubborn crystals, they discovered two completely new forms of carbon.
Diamond is so hard because the carbon atoms inside it are arranged in a tetrahedron-shaped lattice that is immensely strong. In Haverö, the researchers found crystalline carbon arranged in a rhombohedral lattice. This type of diamond was predicted to exist decades ago but had never been seen in nature.
The second substance turned out to be a totally new kind of crystalline carbon, which the researchers call “an intermediate between graphite and diamond”.
Graphite, like diamond, is made up entirely of carbon atoms. However, its atoms are arranged in honeycomb-like sheets. The sheets are only weakly attracted to each other, making it soft and slippery.
When the meteorite entered Earth’s atmosphere, the researchers think pressure shocks and intense heat fused sheets of graphite together, much like the way labs make artificial diamonds.
Unfortunately, the crystals are so small that no one has been able to test the limits of their hardness, nor compare them with the artificial ultra-hard diamonds lonsdaleite and boron nitride.
Autunite
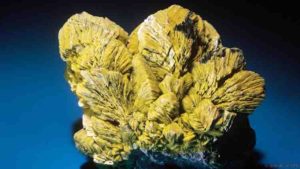
Autunite is a mineral that the big kid in everyone can get excited about. Its tablet-shaped crystals look like lurid yellow-green scales, its uranium content makes it radioactive, and – the icing on the cake of cool – it fluoresces.
When ultraviolet light shines on an autunite crystal, it imparts energy to electrons within the crystal’s uranium atoms. Each excited electron jumps momentarily away from the nucleus of its atom, then falls back.
When the electrons drop back, they release bursts of visible light. The collective effect makes autunite appear to glow green.
Fluorescent minerals stop glowing when the ultraviolet light source is removed. Other minerals are phosphorescent: the electrons remain in an excited state for longer, so phosphorescent minerals continue to glow for a while even after the light is turned off.
Sugar
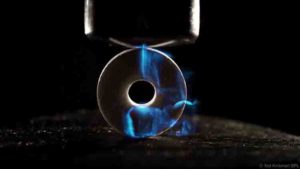
Want to see a crystal glow, but don’t have access to a mineral library? No problem.
Get yourself some sugar cubes or polo mints, go to a pitch-black room, and use the bottom of a glass to smash them to pieces. You should see a fleeting faint blue glow emanate from the sugary treats. This is called triboluminescence.
Literally meaning “rub light”, it was first noted by 17th Century polymath Francis Bacon. Later, Robert Boyle observed that: “hard sugar being nimbly scraped with a knife would afford a sparkling light”.
Centuries later, quite how sugar can be triboluminescent is still a mystery.
Current theories postulate that when sugar crystals are scraped, fractured or crushed, their structural asymmetry encourages tiny piezoelectric fields to form. This separates positive and negative charges within the crystal, and when these charges recombine, a spark flies. Then, nitrogen molecules trapped within the crystals absorb this energy and luminesce, much as they do during a lightning storm.
If that’s true, triboluminescence is almost literally a storm in a teacup.
Biophotonic crystal
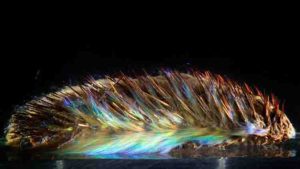
Photonic crystals are tiny repeating structures, each about a billionth of a metre across. They can control and manipulate how light flows.
Depending on the angles of its faces, a photonic crystal will only allow certain wavelengths of light through, and blocks all the others. This determines its colour.
The blocked wavelengths are called “photonic band gaps”. Wavelengths near these band gaps tend to scatter and interfere with one another. This is what creates the vivid colours and striking iridescence of some insects, particularly butterflies and beetles, whose colours appear to change depending on the angle they’re viewed from.
Humans can make simple photonic crystals from synthetic polymers. We use them to create things like reflective coatings for sunglasses.
If we could only duplicate the most complex photonic structures – like those seen in beetles, butterflies, bees and spiders – we could use them to improve everything from fibre-optic technologies to solar cells.
So far, engineers have struggled to build precisely-organised three-dimensional structures on usable scales. However, new research into the way biophotonic crystals take shape in insects offers some promising pointers.
Volcanic ice crystals
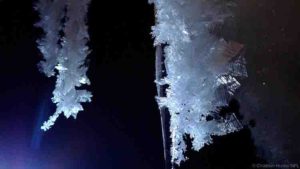
Mount Erebus in Antarctica is the southernmost active volcano in the world. Dotted around its summit is a network of ice caves, which harbour fragile ice formations that occur nowhere else on the planet.
The labyrinth of passages is carved into the snowpack by hot gases from the volcano, which seep out through cracks and fissures in the underlying rock. Within the caves, the warm, steamy air from the volcano hits the frigid walls, whereupon the moisture freezes into intricate, feathery shapes, guided by the air currents.
The resulting crystals look like clusters of snowflakes.
Craig Cary of the University of Waikato in New Zealand has spent time in the caves and was struck by the delicacy of the ice formations. “They hang down maybe half a metre from the ice ceiling, and it only takes the wind generated by a slowly passing body underneath to cause them to fall,” he says.
The crystals are an example of hoarfrost, which is formed when moisture condenses and freezes directly onto objects.
When ice grows slowly, as it does in liquid water, it forms solid hexagonal crystals. But if the water vapour is particularly thick, and there is space to grow, the ice will instead grow into the hexagonally symmetrical branching forms seen at Erebus.
Note: The above post is reprinted from materials provided by BBC Earth. The original article was written by Ceri Perkins.