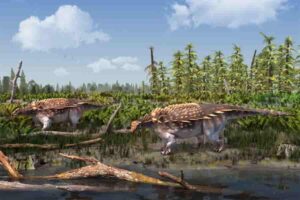
A new armoured dinosaur, known as an ankylosaur, has been described and named for Prof Paul Barrett of the Natural History Museum.
Vectipelta barretti was discovered in the Wessex formation on the Isle of Wight and represents the first armoured dinosaur from the dinosaur Isle to be described in 142 years.
Lead author Stuart Pond explained the importance of this find, ‘This is an important specimen because it sheds light on ankylosaur diversity within the Wessex formation and Early Cretaceous England.
‘For virtually 142 years, all ankylosaur remains from the Isle of Wight have been assigned to Polacanthus foxii, a famous dinosaur from the island, now all of those finds need to be revisited because we’ve described this new species.’
The new species differs from Polacanthus foxii, previously the only known ankylosaur from the Isle of Wight, in several key characteristics. The fossilized remains show differences in the neck and back vertebrae, a very different structure to the pelvis and more blade-like spiked armour.
The researchers used phylogenetic analysis to work out the relationships between different ankylosaurs and discovered that they are not actually very closely related. In fact, Vectipelta was found to be most closely related to some Chinese ankylosaurs, suggesting dinosaurs moved freely from Asia to Europe in the Early Cretaceous.
Vectipelta barretti would have been roaming the earth during the Early Cretaceous, a time for which fossil remains are rare worldwide. This has led some to suggest that a mass extinction occurred at the end of the Jurassic, which makes the understanding of dinosaur diversity at this time crucial to understanding if such an event occurred and how life recovered. With rocks from this time mostly absent in North America, the Wessex Formation and the Isle of Wight are hugely important areas in answering these questions.
At the time the Isle of Wight would have had a climate similar to that of the Mediterranean and was a flood plain covered by a large meandering river system. Floods would have washed organic material such as plants, logs and even dinosaur bodies together and, as waters receded, this organic matter would have been isolated in ponds on the floodplain that eventually dried out and were buried in the clay soil, preserving this organic material as the fossils we find today.
On naming the new dinosaur for Prof Paul Barrett of the Natural History Museum, senior author Dr Susannah Maidment said, ‘Myself and some of the other authors on this study have been mentored or supervised by Paul for most of our careers, and it was notable to us that Paul hadn’t had a dinosaur named after him yet. He’s hugely influential in in vertebrate palaeontology, and he’s a world-leading authority on dinosaurs.
‘We really wanted to thank him for his support and mentorship, so we decided to name a, slow-moving, spikey organism after him.’
Prof Paul Barrett has worked at the Natural History Museum, London for 20 years and in that time has published an impressive 220 scientific papers. He has also supervised 31 PhD students and mentored many others, encouraging a whole new generation of palaeontologists.
Of the honour Prof Barrett said, ‘I’m flattered and absolutely delighted to have been recognised in this way, not least as the first paper I ever wrote was also on an armoured dinosaur in the NHM collections. I’m sure that any physical resemblance is purely accidental.’
The team are optimistic that more species will be discovered in the area in the future. Dr Maidment concluded, ‘We have new iguanodontians that we are lining up, to be prepped and to be studied. I think we have at least two new taxa in the collections. With regards to ankylosaurs, they are somewhat rarer, so I think we need to keep our eyes peeled.’
The new dinosaur is part of the internationally important collection held at Dinosaur Isle Museum, operated by the Isle of Wight Council. Parts of the dinosaur will be on display at the museum for the school holidays. The bones have been cleaned from their surrounding matrix by staff and volunteers at the museum which has made the research possible.
Reference:
Stuart Pond, Sarah-Jane Strachan, Thomas J. Raven, Martin I. Simpson, Kirsty Morgan, Susannah C. R. Maidment. Vectipelta barretti, a new ankylosaurian dinosaur from the Lower Cretaceous Wessex Formation of the Isle of Wight, UK. Journal of Systematic Palaeontology, 2023; 21 (1) DOI: 10.1080/14772019.2023.2210577
Note: The above post is reprinted from materials provided by Taylor & Francis Group.