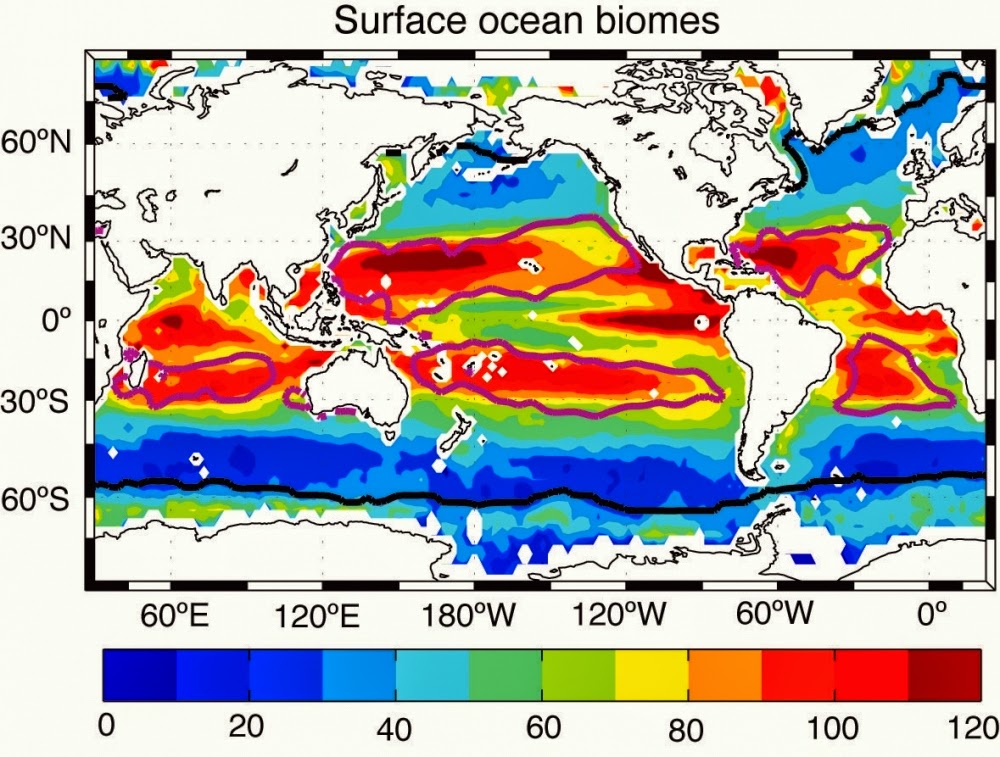
The ocean is a complex ecosystem. The ocean carbon cycle is governed by the relationship among carbon, nutrients and oxygen, and the ratio between certain elements is key to understanding ocean respiration.
Phytoplankton — photosynthesizing microscopic organisms inhabiting the upper sunlit layer of the ocean — play an important role in governing carbon, nutrients and oxygen cycles. A new study by UC Santa Barbara’s Timothy DeVries and Curtis Deutsch of the University of Washington reveals a threefold variation across latitudes in the ratio of oxygen consumed to phosphorus released during organic matter respiration. The findings, which appear today in the journal Nature Geoscience, demonstrate how climate change might affect the ratio of carbon, oxygen and nutrients in the subsurface ocean.
Just like trees and plants on Earth’s surface, phytoplankton take up nutrients and carbon, which are processed and released as organic matter that sinks to the ocean’s subsurface. In this study, DeVries and Deutsch focused primarily on the depth of 200 meters, which is below the photic zone. With no light no photosynthesis can occur. Once again mimicking the biological processes that take place on Earth’s surface, marine microbes in the ocean consume the organic matter and use oxygen to respire it.
The scientists estimated how much oxygen the microbes used per unit of phosphorus they have consumed, expressed as a ratio, O2:P. “The interesting thing we see is that this ratio varies a great deal,” said DeVries, a newly appointed assistant professor in UCSB’s Department of Geography and at the campus’s Earth Research Institute.
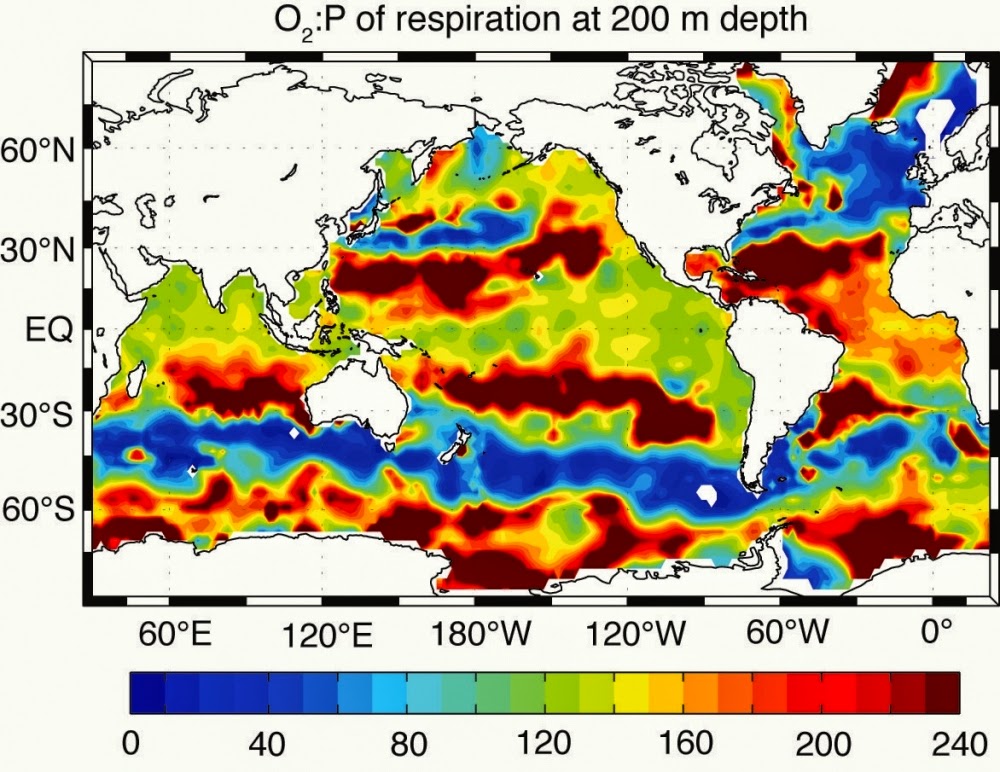
“We’re not able to determine what varies the ratio, but just based on basic chemistry and biology, we know that it’s probably in large part due to variations in the carbon-to-phosphorus ratio of the organic matter that the microbes are consuming,” DeVries added. “So a high C:P ratio would correlate with a high O2:P ratio of respiration. The more carbon there is in the organic matter, the more oxygen it takes to respire it.
We’re quite confident that the O2:P ratio of microbial respiration that we’re seeing correlates with the C:P ratio of the phytoplankton. There have been measurements of this in the surface ocean and those measurements correlate to what we’re seeing in the subsurface.”
Such ratios, known as stoichiometry, describe the relative quantities of reactants and products in chemical reactions. The ocean circulation model and global climatologies of oxygen and phosphate used by DeVries and Deutsch allowed them to detect strong regional variabilities in the ratios of respired oxygen and phosphorus in the subsurface ocean. Previous analyses were unable to detect these variations because of the highly simplified ways they represented ocean mixing processes. In addition, the researchers said that ratio variations show close correlation with environmental conditions in the surface ocean.
Central to the team’s modeling results is how these spatial variations correlate with different ocean surface biomes or ecosystems. According to DeVries, some surface areas of the ocean contain few nutrients but an abundance of sunlight; other areas manifest the opposite combination: low light and plenty of nutrients.
“One of the reasons this is important is that in an ocean growing warmer under climate change, we expect an increase in regions of the ocean that have more light and are nutrient poor,” DeVries said. “Based on these patterns, we expect that will shift the phytoplankton community to a higher C:P ratio. If there is a shift toward this regime, there’s going to be more carbon stored in the ocean than the corresponding situation in which there is no shift.”
That is actually a good thing, DeVries noted. Phytoplankton taking up more carbon because they are exposed to more light and fewer nutrients could help offset the predicted slowdown of the ocean’s uptake of carbon dioxide from the atmosphere. “As the Earth’s climate warms, it will cause the oceans to stratify and reduce their capacity to take up CO2, causing warming to accelerate even faster,” DeVries continued. “This shift in C:P ratios that we predict could slow the acceleration slightly, but not halt it entirely.”
A related issue is how oceanic oxygen levels will change in the future. “As the ocean warms and the water holds less oxygen, low-oxygen regions are going to expand, and this will put a great deal of stress on marine animals,” DeVries concluded. “How much they expand and whether we can predict the extent is going to depend on the types of stoichiometric variability we see in this study.”
Reference:
Tim DeVries, Curtis Deutsch. Large-scale variations in the stoichiometry of marine organic matter respiration. Nature Geoscience, 2014; DOI: 10.1038/ngeo2300
Note : The above story is based on materials provided by University of California – Santa Barbara. The original article was written by Julie Cohen.