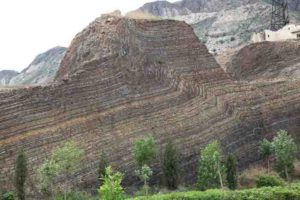
The first photosynthetic oxygen-producing organisms on Earth were cyanobacteria. Their evolution dramatically changed the Earth allowing oxygen to accumulate into the atmosphere for the first time and further allowing the evolution of oxygen-utilizing organisms including eukaryotes. Eukaryotes include animals, but also algae, a broad group of photosynthetic oxygen-producing organisms that now dominate photosynthesis in the modern oceans. When, however, did algae begin to occupy marine ecosystems and compete with cyanobacteria as important phototrophic organisms?
In a new study Zhang et al use the molecular remains of ancient algae (so-called biomarkers) to show that algae occupied an important role in marine ecosystems 1400 million years ago, some 600 million years earlier than previously recognized.
The specific biomarkers explored by Zhang et al are a group of sterane molecules derived from sterols that are prominent components of cell membranes in eukaryotic organisms. A particular difficulty in analyzing for ancient steranes is that samples are easily contaminated with steranes from other sources. The sources of contamination range from steranes introduced during the sampling, transport and processing of the samples, to geological contamination of steranes as fluids have flow through the rocks.
Zhang et al carefully controlled for each of the sources of contamination and found, as have others, that no steranes were liberated when using standard protocols to extract biomarkers from such ancient rocks, in this case the 1400 million-year-old Xiamaling Formation in North China.
However, Shuichang Zhang, the lead author of the study speculated that “There is some fossil evidence for eukaryotic algae 1400 million years ago, or even earlier, so we wondered whether any steranes in these rocks might be more tightly bound to the kerogens and not easily released during standard biomarker extraction.” Therefore, Zhang et al utilized a stepwise heating protocol where samples were slowly heated in gold tubes in 9 steps from 300°C to 490°C. The organic molecules liberated in each of the nine steps were extracted and steranes indicating the presence of both red and green algae were liberated, especially at the higher temperatures.
Zhang continues “Many will be concerned that the steranes we found were a product of some kind of contamination. We were also worried about this, but we ran in parallel samples that have been heated to high temperatures during their geologic history and that, therefore, contained no biomarkers. We found no steranes in these. This means that our protocols were clean, and we are therefore confident that the steranes we found were indigenous to the rock.”
It’s still not completely clear why the steranes were so tightly bound to the kerogen and not released during standard protocols. But, the findings of Zhang et al. show that both green and red algal groups were present in marine ecosystems by 1400 million years ago. This is 600 million years earlier than evident from previous biomarker studies. This work shows that the red and green algal lineages had certainly evolved by 1400 million years ago, and this should be a useful constraint in timing the overall history of eukaryote evolution. This work also shows that at least some ancient marine ecosystems functioned more similarly to modern ecosystems than previously thought, at least with respect to the types of photosynthetic organisms producing organic matter. This means furthermore that there was sufficient nutrients and oxygen available to drive the presence of algae-containing ecosystems.
Professor Don Canfield, Nordic Center for Earth Evolution, University of Southern Denmark, a co-author on the study adds: “We hope that our study will inspire others to utilize similar techniques to better unravel the full history of eukaryote evolution through geologic time.”
Reference:
Shuichang Zhang, Jin Su, Sihong Ma, Huajian Wang, Xiaomei Wang, Kun He, Huitong Wang, Donald E. Canfield. Eukaryotic red and green algae populated the tropical ocean 1400 million years ago. Precambrian Research, 2021; 357: 106166 DOI: 10.1016/j.precamres.2021.106166
Note: The above post is reprinted from materials provided by University of Southern Denmark. Original written by Birgitte Svennevig.