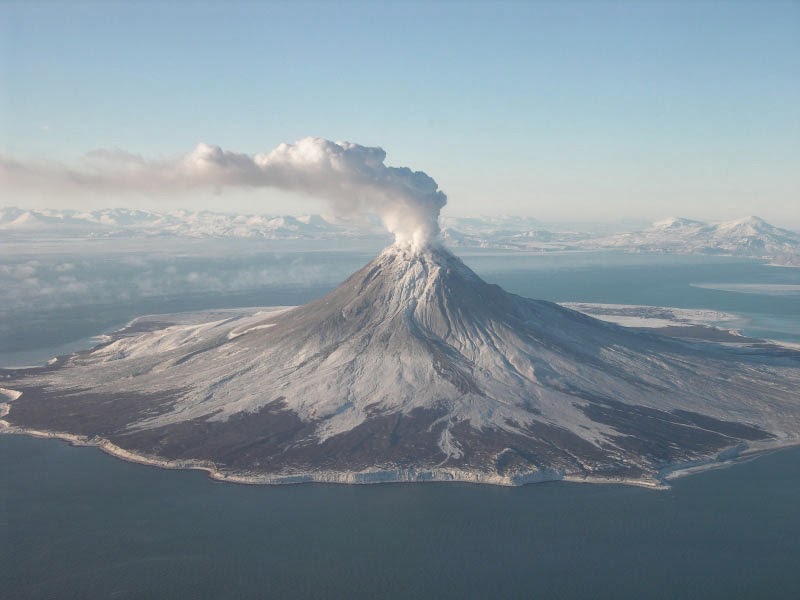
New research in Geophysical Research Letters examines earthquake swarms caused by mounting volcanic pressure which may signal an imminent eruption. The research team studied Augustine Volcano in Alaska which erupted in 2006 and found that precursory earthquakes were caused by a block in the lava flow.
36 hours before the first magmatic explosions, a swarm of 54 earthquakes was detected across the 13-station seismic network on Augustine Island. By analyzing the resulting seismic waves, the authors found that the earthquakes were being triggered from sources within the volcano’s magma conduit.
“Our article talks about a special type of volcanic earthquake that we think is caused by lava breaking, something that usually can’t happen because lava is supposed to flow more like a liquid, rather than crack like a piece of rock,” said Dr. Helena Buurman from the University of Alaska Fairbanks. “Much like breaking a piece of chewing gum by stretching it really fast, lab tests show that hot lava can break when stretched quickly enough under certain pressures like those that you might find in the conduit of a volcano. ”
The authors found that over the course of the two hour swarm, the earthquakes’ focus moved 35 meters deeper down into the magma conduit, an indication that the conduit was becoming clogged. The resulting buildup of pressure may have contributed to the explosive eruption the next day.
“We think that these earthquakes happened within the lava that was just beginning to erupt at the top of Augustine. The earthquakes show that the lava flow was grinding to a halt and plugging up the system. This caused pressure to build up from below, and resulted in a series of large explosions 36 hours later,” concluded Dr. Buurman. “We believe that these types of earthquakes can be used to signal that a volcano is becoming pressurized and getting ready to explode, giving scientists time to alert the public of an imminent eruption.”
Note : The above story is based on materials provided by Wiley