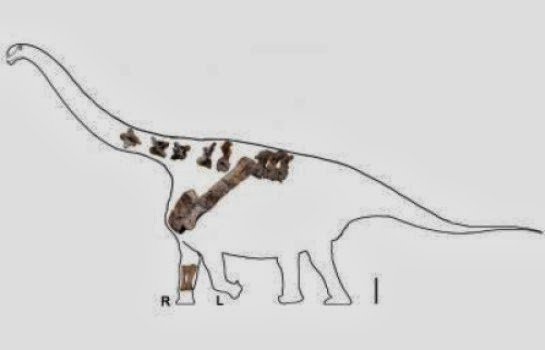
A team led by University of Pennsylvania paleontologists has characterized a new dinosaur based on fossil remains found in northwestern China. The species, a plant-eating sauropod named Yongjinglong datangi, roamed during the Early Cretaceous period, more than 100 million years ago. This sauropod belonged to a group known as Titanosauria, members of which were among the largest living creatures to ever walk the earth.
At roughly 50-60 feet long, the Yongjinglong individual discovered was a medium-sized Titanosaur. Anatomical evidence, however, points to it being a juvenile; adults may have been larger.
The find, reported in the journal PLOS ONE, helps clarify relationships among several sauropod species that have been found in the last few decades in China and elsewhere. Its features suggest that Yongjinglong is among the most derived, or evolutionarily advanced, of the Titanosaurs yet discovered from Asia.
Doctoral student Liguo Li and professor Peter Dodson, who have affiliations in both the School of Veterinary Medicine’s Department of Animal Biology and the School of Arts and Sciences’ Department of Earth and Environmental Science, led the work. They partnered with Hailu You, a former student of Dodson’s, who now works at the Chinese Academy of Sciences’ Institute of Vertebrate Paleontology and Paleoanthropology, and Daqing Li of the Gansu Geological Museum in Lanzhou, China.
Until very recently, the United States was the epicenter for dinosaur diversity, but China surpassed the U.S. in 2007 in terms of species found. This latest discovery was made in the southeastern Lanzhou-Minhe Basin of China’s Gansu Province, about an hour’s drive from the province’s capital, Lanzhou. Two other Titanosaurs from the same period, Huanghetitan liujiaxiaensis and Daxiatitan binglingi, were discovered within the last decade in a valley one kilometer from the Yongjinglong fossils.
“As recently as 1997 only a handful of dinosaurs were known from Gansu,” Dodson said. “Now it’s one of the leading areas of China. This dinosaur is one more of the treasures of Gansu.”
During a trip to Gansu, Liguo Li was invited to study the remains, which had been in storage since being unearthed in 2008. They consisted of three teeth, eight vertebrae, the left shoulder blade, and the right radius and ulna.
The anatomical features of the bones bear some resemblance to another Titanosaur that had been discovered by paleontologists in China in 1929, named Euhelopus zdanskyi. But the team was able to identify a number of unique characteristics.
“The shoulder blade was very long, nearly 2 meters, with sides that were nearly parallel, unlike many other Titanosaurs whose scapulae bow outward,” Li said.
The scapula was so long, indeed, that it did not appear to fit in the animal’s body cavity if placed in a horizontal or vertical orientation, as is the case with other dinosaurs. Instead, Li and colleagues suggest the bone must have been oriented at an angle of 50 degrees from the horizontal.
In addition, an unfused portion of the shoulder blade indicated to the researchers that the animal under investigation was a juvenile or subadult.
“The scapula and coracoid aren’t fused here,” Li said. “It is open, leaving potential for growth.”
Thus, a full-grown adult might be larger than this 50-60 foot long individual. Future finds may help elucidate just how much larger, the researchers noted.
The ulna and radius were well preserved, enough so that the researchers could identify grooves and ridges they believe correspond with the locations of muscle attachments in the dinosaur’s leg.
The researchers were also able to draw evidence about the dinosaur’s relationship to other species from the vertebrae, one of which was from the neck and the other seven from the trunk. Notably, the vertebrae had large cavities in the interior that the team believes provided space for air sacs in the dinosaur’s body.
“These spaces are unusually large in this species,” Dodson said. “It’s believed that dinosaurs, like birds, had air sacs in their trunk, abdominal cavity and neck as a way of lightening the body.”
In addition, the longest tooth they found was nearly 15 centimeters long. Another shorter tooth contained unique characteristics, including two “buttresses,” or bony ridges, on the internal side, while Euhelopus had only one buttress on its teeth.
To gain a sense of where Yongjinglong sits on the family tree of sauropods, the researchers were able to compare its characteristics with specimens from elsewhere in China, as well as from Africa, South America and the U.S.
“We used standard paleontological techniques to compare it with phylogenies based on other specimens,” Dodson said. “It is definitely much more derived than Euhelopus and shows close similarities to derived species from South America.”
Not only does the discovery point to the fact that Titanosaurs encompass a diverse group of dinosaurs, but it also supports the growing consensus that sauropods were a dominant group in the Early Cretaceous — a view that U.S. specimens alone could not confirm.
“Based on U.S. fossils, it was once thought that sauropods dominated herbivorous dinosaur fauna during the Jurassic but became almost extinct during the Cretaceous,” Dodson said. “We now realize that, in other parts of the world, particularly in South America and Asia, sauropod dinosaurs continued to flourish in the Cretaceous, so the thought that they were minor components is no longer a tenable view.”
Note : The above story is based on materials provided by University of Pennsylvania. Note: Materials may be edited for content and length.