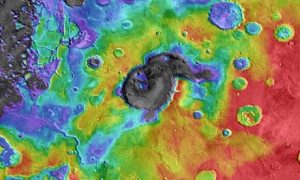
Ancient Mars was home to giant volcanoes capable of eruptions a thousand times more powerful than the one that shook Mount St Helens in 1980, scientists have said.
The finding raises fresh questions about conditions on Mars in its early years, a time when scientists believe the planet was much more Earth-like, with a thick atmosphere, warmer temperatures and water on its surface.
Major volcanic eruptions may well have triggered climate shifts that toggled Martian temperatures between cold spells when ash blocked out the sunlight and heat waves when greenhouse gases filled the skies, according to scientists.
Supervolcanoes may have made it more difficult for life to evolve on the planet’s surface, but underground steam vents and the release of water into the atmosphere also could have created niches for microbes to thrive, said geologist Joseph Michalski of the Planetary Science Institute in Tucson, Arizona.
The discovery of supervolcanoes on Mars comes from analysis of images from a quartet of Mars orbiters over the past 15 years.
These types of volcanoes, also known as “caldera” volcanoes, are ancient, collapsed structures rather than steep, cone-shaped or domed mountains like Olympus Mons on Mars, a so-called shield volcano that stands nearly three times taller than Mount Everest, the highest peak on Earth.
“We know a lot about the volcanic history of Mars over the last 3bn to 3.5bn years, but that still leaves about 1bn years before that over which we don’t really know anything about volcanism,” Michalski told Reuters.
Some scientists theorised that the oldest Martian volcanoes had eroded away, but the new findings suggest a different kind of volcano existed long ago.
“If early Mars saw a lot more explosive volcanism, then the features that are left from that don’t look like those shield volcanoes. That’s maybe why we didn’t see them,” Michalski said.
Scientists say supervolcanoes erupt with about 1,000 times the force of typical volcanoes like Mount St Helens in Washington state. The eruption of Mount St Helens in 1980 blasted the top off the mountain, killed 57 people and, according to the US Geological Survey, shot ash, steam, water and debris about 24,000 metres (80,000ft) into the air.
Evidence of past supervolcanoes on Earth has been erased by plate tectonics and other geologic activities.
Michalski was actually studying Martian impact craters, not looking for volcanoes.
“We made the discovery by accident,” he said. “As I went through [the images] of this one region, I found a number of them that were simply not impact craters,” he said.
“One was clearly a volcano. … It is quite possible there are many more of these,” Michalski added.
Because the emission of gases from volcanoes helps create a planet’s atmosphere, understanding the volcanic history of Mars is crucial to figuring out what the planet – the fourth from the Sun – was like in its early years.
Additional evidence may come from Nasa’s Mars Curiosity rover, which is heading toward a 3-mile-(5-km)-high mound of deposits called Mount Sharp.
The rover touched down inside a giant impact basin near the planet’s equator in August 2012 to assess if Mars ever had the chemistry and environment to support and preserve microbial life.
“There are thousands of layers of rocks in Mount Sharp and they contain a long record of geologic history,” Michalski said.
“There could be interlayered rocks that are ash beds, and we predict that and we hope that the rover can test it,” he said.
The research appears in the journal Nature.
Note: The above story is based on materials provided by Reuters in Houston