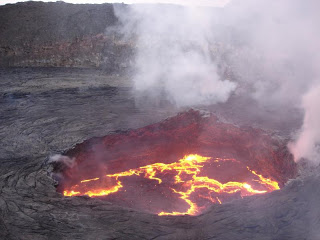
Volcanic ‘Plumbing Systems’ Exposed
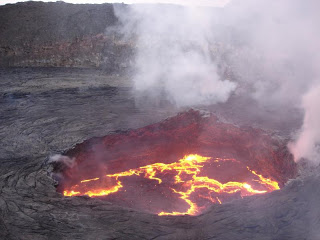
Expedition to Undersea Mountain Yields New Information About Sub-Seafloor Structure
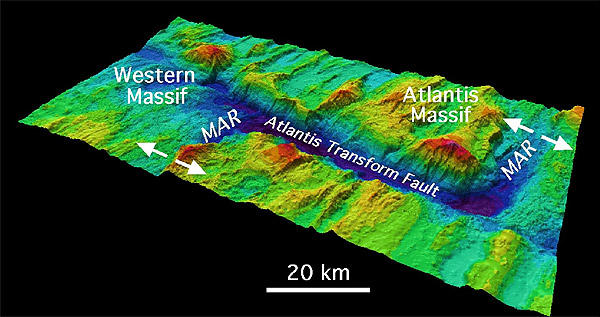
Global Sea Level Likely to Rise as Much as 70 Feet for Future Generations
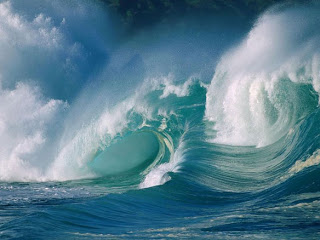
Even if humankind manages to limit global warming to 2 degrees C (3.6 degrees F), as the Intergovernmental Panel on Climate Change recommends, future generations will have to deal with sea levels 12 to 22 meters (40 to 70 feet) higher than at present, according to research published in the journal Geology.
New Theory On Formation of Oldest Continents
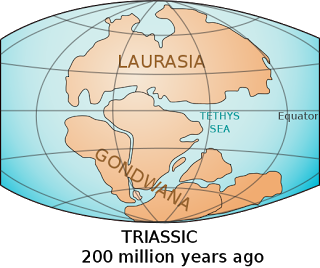
Following trace elements:
The oceanic crust did not have to descend:
Flying Through a Geomagnetic Storm
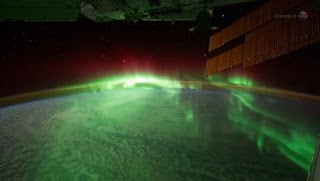
Mapping the Moho With GOCE
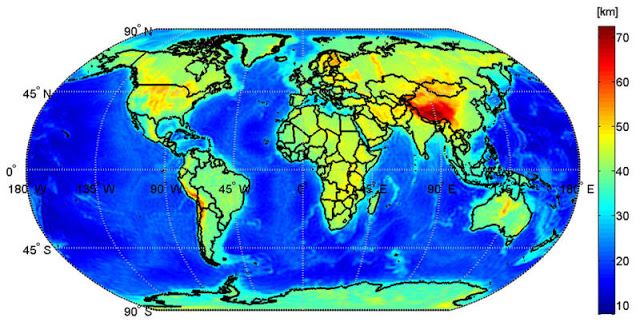
Internal Structure of the Moon
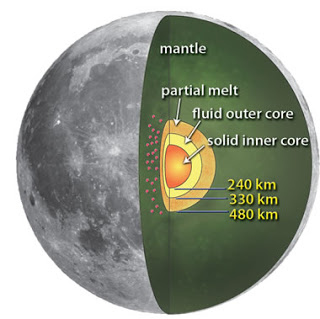
Apollo Data Revealed Moon’s Internal Structure:
NASA deployed the frist seismographs on the moon as part of the Apollo Mission in 1969. These seismographs collected data and enabled researchers to determine that the moon’s structure consisted of a thin crust of about 65 kilometers, a mantle about 100 kilometers thick and a core with a radius of about 500 kilometers. At that time seismic data processing was not advanced enough to determine the characteristics of the core.
NASA researchers have recently applied state-of-the-art seismological techniques applied to the Apollo-era data and discovered that the moon probably has a core that is very similar to Earth’s.
Moon’s Formation and Magnetic Field :
The team’s findings suggest the moon possesses a solid, iron-rich inner core with a radius of nearly 150 miles and a fluid, primarily liquid-iron outer core with a radius of roughly 205 miles. Where it differs from Earth is a partially molten boundary layer around the core estimated to have a radius of nearly 300 miles. The research indicates the core contains a small percentage of light elements such as sulfur, echoing new seismology research on Earth that suggests the presence of light elements — such as sulfur and oxygen — in a layer around our own core.
Data from Apollo-Era Seismometers :
Comparison with Apollo-Era Results :
New Data From the GRAIL Mission : Future NASA missions will help gather more detailed data. The Gravity Recovery and Interior Laboratory, or GRAIL, is a NASA Discovery-class mission set to launch this year. The mission consists of twin spacecraft that will enter tandem orbits around the moon for several months to measure the gravity field in unprecedented detail. The mission also will answer longstanding questions about Earth’s moon and provide scientists a better understanding of the satellite from crust to core, revealing subsurface structures and, indirectly, its thermal history.
The Research Team :
In addition to Weber, the team consisted of scientists from Marshall; Arizona State University; the University of California at Santa Cruz; and the Institut de Physique du Globe de Paris in France. Their findings are published in the online edition of the journal Science.
NASA and other space agencies have been studying concepts to establish an International Lunar Network — a robotic set of geophysical monitoring stations on the moon — as part of efforts to coordinate international missions during the coming decade
Note : Republished from a January, 2011 press release by NASA.
New Research Supports Theory of Extraterrestrial Impact
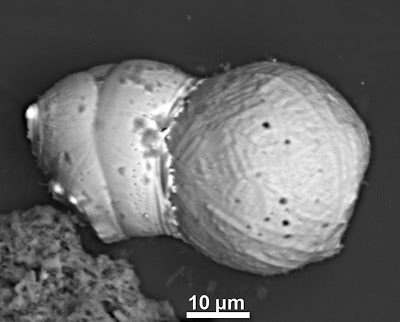
Contamination of La Selva geothermal system in Girona, Spain
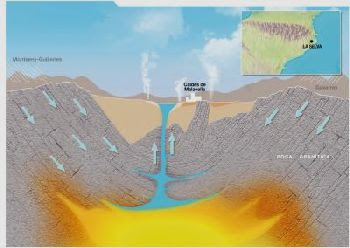
The groundwater in La Selva (Girona, Spain) area show high levels of arsenic, antimony and other polluting elements. The area’s geothermal system, where hot and cold groundwater flow naturally, are the cause of this situation, according to a study that researchers from the Polytechnic University of Catalonia (UPC) and the University of Barcelona (UB) have published in the journal Geothermics.
Thickest Parts of Arctic Ice Cap Melting Faster
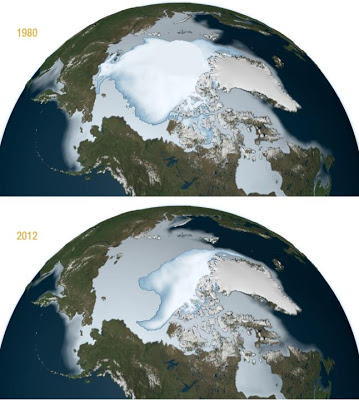
Albite
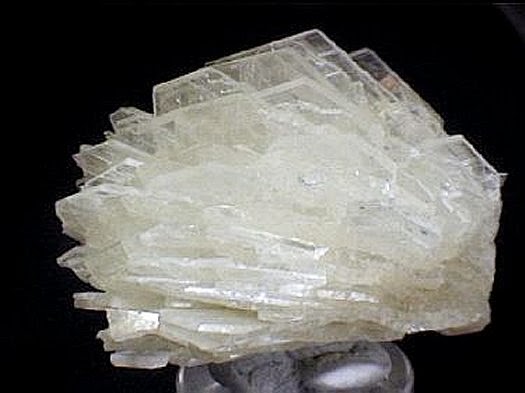
Chemical Formula: NaAlSi3O8
Locality: Finnbo, Falun, Dalarna, Sweden. Bourg d’Oisans and Isere, France.
Name Origin: From the Latin, albus, in allusion to the common color.
Albite is a plagioclase feldspar mineral. It is the sodium endmember of the plagioclase solid solution series. As such it represents a plagioclase with less than 10% anorthite content. The pure albite endmember has the formula NaAlSi3O8. It is a tectosilicate. Its color is usually pure white, hence its name from Latin albus. It is a common constituent in felsic rocks.
Albite crystallizes with triclinic pinacoidal forms. Its specific gravity is about 2.62 and it has a Mohs hardness of 6 – 6.5. Albite almost always exhibits crystal twinning often as minute parallel striations on the crystal face. Albite often occurs as fine parallel segregations alternating with pink microcline in perthite as a result of exolution on cooling.
It occurs in granitic and pegmatite masses, in some hydrothermal vein deposits and forms part of the typical greenschist metamorphic facies for rocks of originally basaltic composition.
It was first reported in 1815 for an occurrence in Finnbo, Falun, Dalarna, Sweden.
Physical Properties
Cleavage: {001} Perfect, {010} Good
Color: White, Gray, Greenish gray, Bluish green, Gray.
Density: 2.61 – 2.63, Average = 2.62
Diaphaneity: Transparent to translucent to subtranslucent
Fracture: Uneven – Flat surfaces (not cleavage) fractured in an uneven pattern.
Hardness: 7 – Quartz
Luminescence: Fluorescent, Short UV=herry-red blue, Long UV=white.
Luster: Vitreous (Glassy)
Streak: white
Photos:
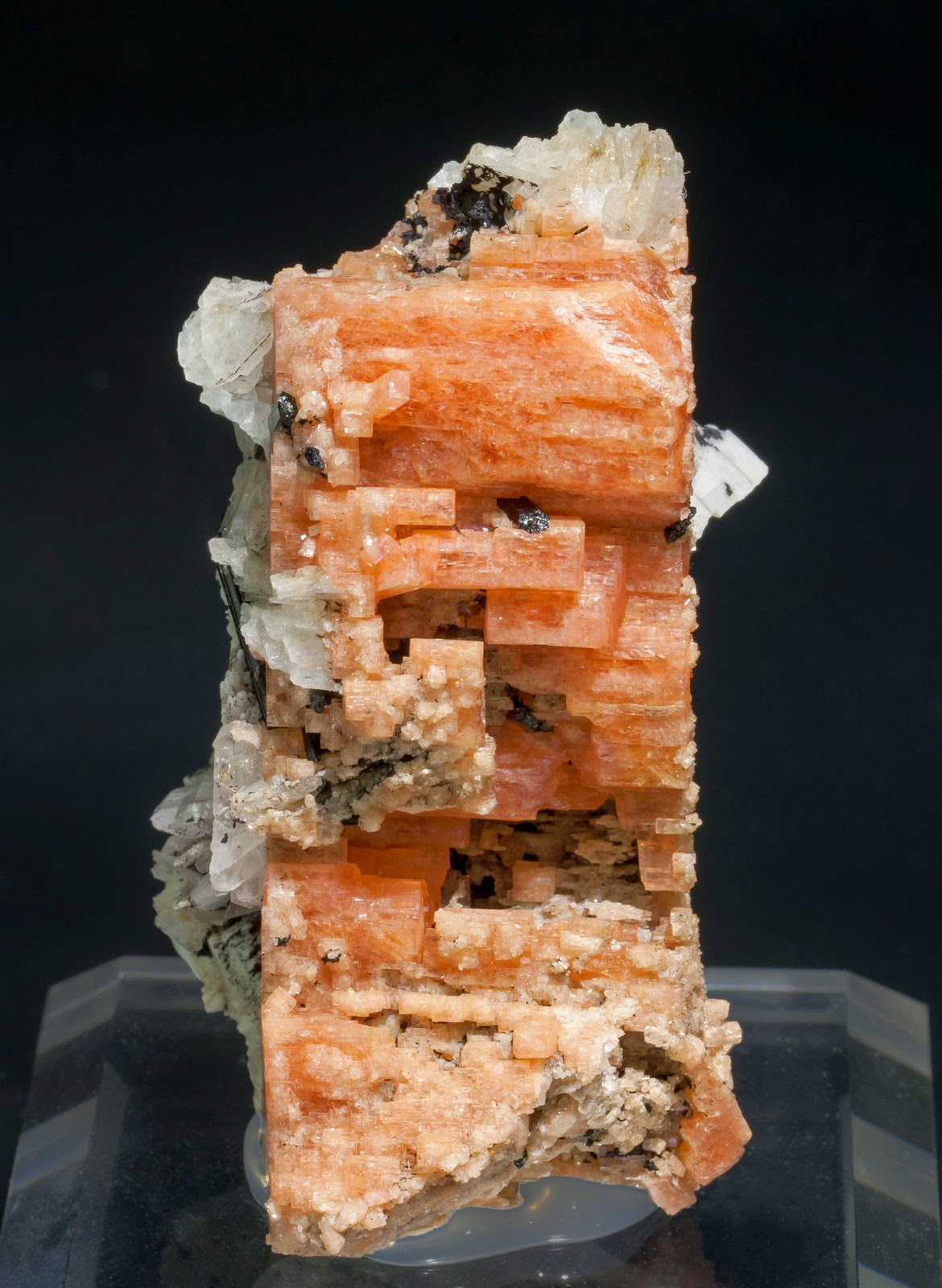
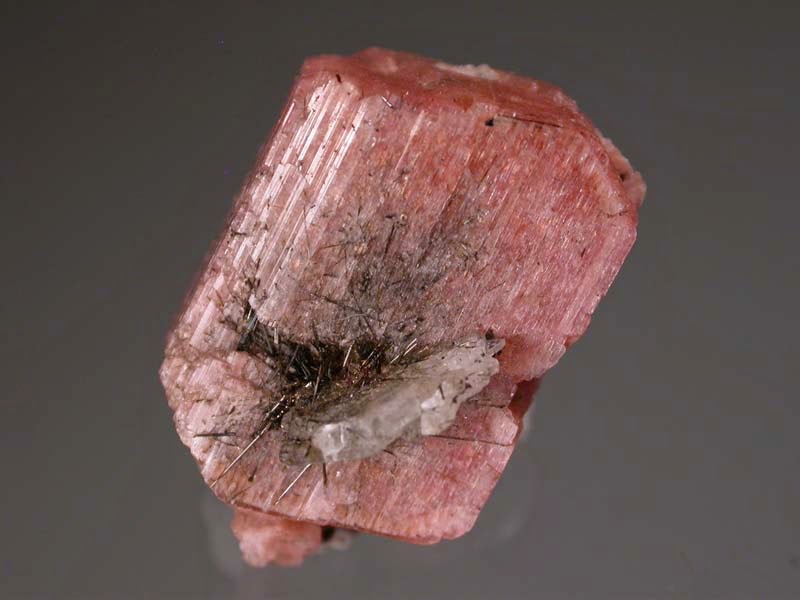
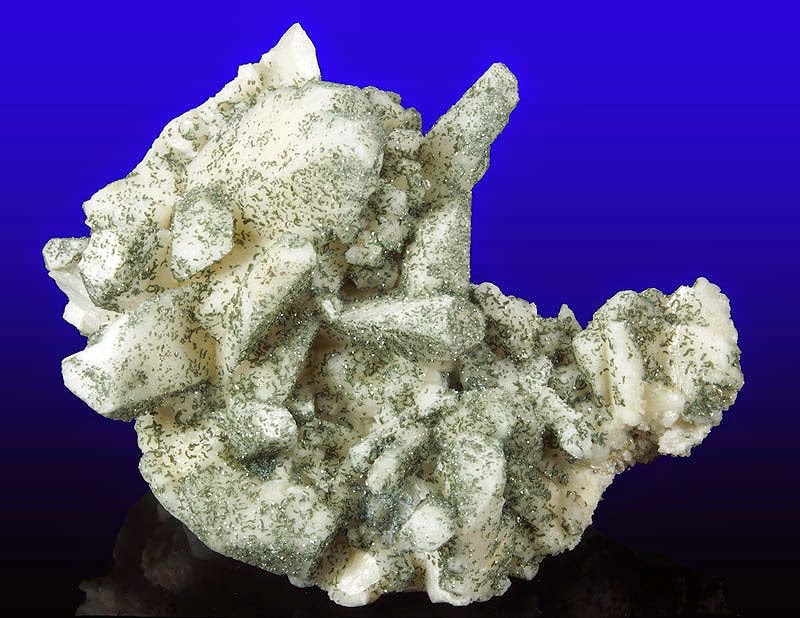
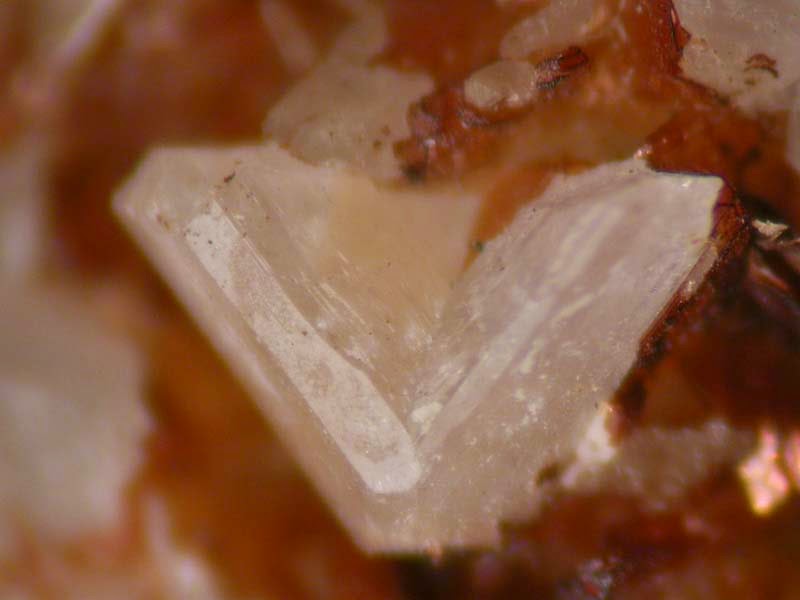
Salty Soil Can Suck Water out of Atmosphere: Could It Happen On Mars?
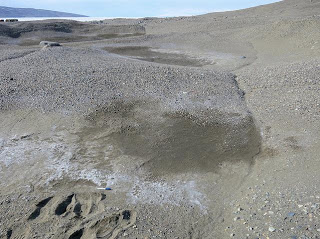
When Continents Collide: New Twist to 50-Million-Year-Old Tale
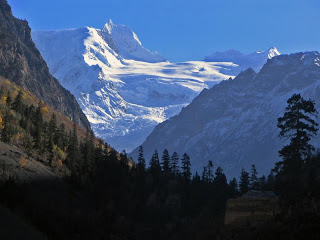
And what will cause it to stop?
Volcanoes Deliver Two Flavors of Water
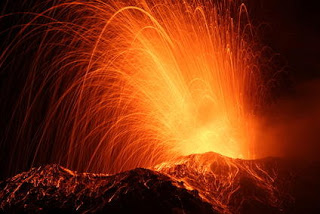
Erosional Origin of Linear Dunes On Earth and Saturn’s Moon Titan
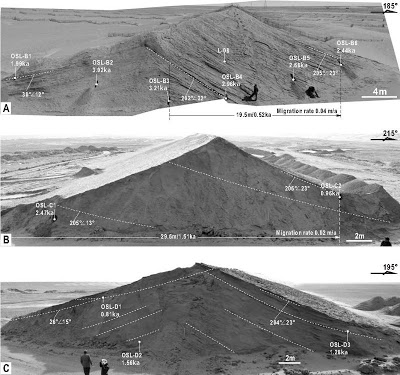
Building Blocks of Early Earth Survived Collision That Created Moon
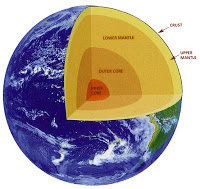
Unexpected new findings by a University of Maryland team of geochemists show that some portions of Earth’s mantle (the rocky layer between Earth’s metallic core and crust) formed when the planet was much smaller than it is now, and that some of this early-formed mantle survived Earth’s turbulent formation, including a collision with another planet-sized body that many scientists believe led to the creation of the Moon.
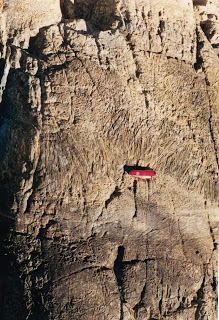
An Isotopic Signature
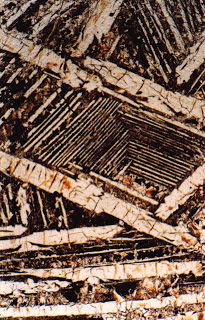
Higher Precision Yields New Findings, Insights
“These findings indicate that the Earth’s mantle has never been completely melted and homogenized, and that convective mixing of the mantle, even while Earth was growing, was evidently very sluggish,” says Walker. “Many questions remain. The rocks we studied are 2.8 billion years old. We don’t know whether the portion of the Earth with this unusual isotopic composition or signature can be found in much younger rocks. We plan to analyze some modern volcanic rocks in the near future to assess this.”
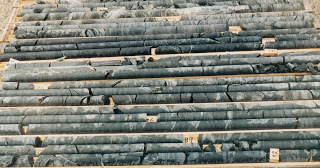
Photo Credit – Igor Puchtel, UMD
300-Million-Year-Old Forest Discovered Preserved in Volcanic Ash
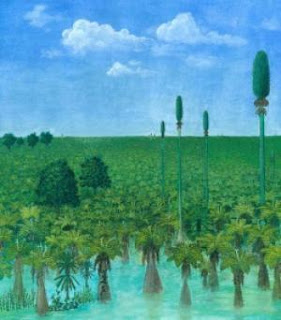
Acanthite
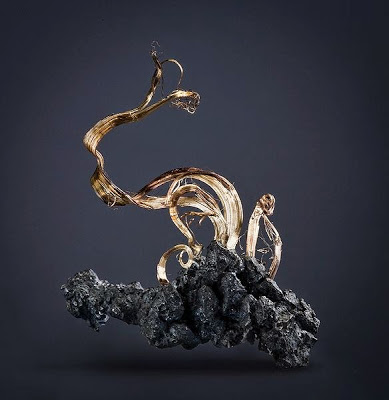
Chemical Formula: Ag2S
Locality: Freiberg, Schneeberg, Annaberg, Germany.
Name Origin: From the Greek, akanta, meaning “arrow.” After the Latin, argentum, meaning “silver”. Argentite is stable above 179 C. Acanthite is stable below 179 deg. C.
Acanthite, Ag2S, crystallizes in the monoclinic system and is the stable form of silver sulfide below 173 °C. Argentite is the stable form above that temperature. As argentite cools below that temperature its cubic form is distorted to the monoclinic form of acanthite. Below 173 °C acanthite forms directly. Acanthite is the only stable form in normal air temperature.
Occurrence
Acanthite is a common silver mineral in moderately low-temperature hydrothermal veins and in zones of supergene enrichment. It occurs in association with native silver, pyrargyrite, proustite, polybasite, stephanite, aguilarite, galena, chalcopyrite, sphalerite, calcite and quartz.
Acanthite was first described in 1855 for an occurrence in the Jáchymov (St Joachimsthal) District, Krušné Hory Mts (Erzgebirge), Karlovy Vary Region, Bohemia, Czech Republic. The name is from the Greek “akantha” meaning thorn or arrow, in reference to its crystal shape.
Physical Properties
Cleavage: {001} Poor, {110} Poor
Color: Lead gray, Gray, Iron black.
Density: 7.2 – 7.4, Average = 7.3
Diaphaneity: Opaque
Fracture: Sectile – Curved shavings or scrapings produced by a knife blade, (e.g. graphite).
Hardness: 2-2.5 – Gypsum-Finger Nail
Luminescence: Non-fluorescent.
Luster: Metallic
Magnetism: Nonmagnetic
Streak: shining black
Photos:
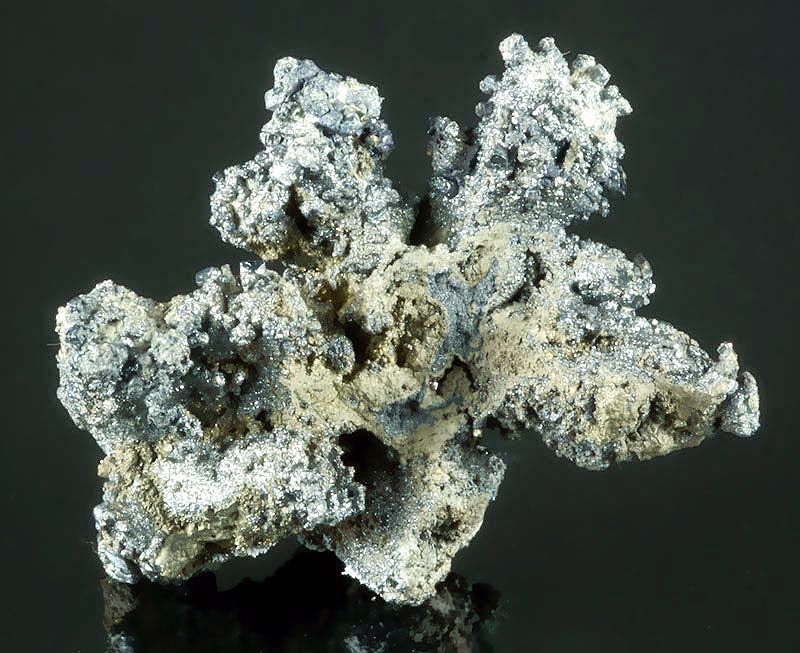
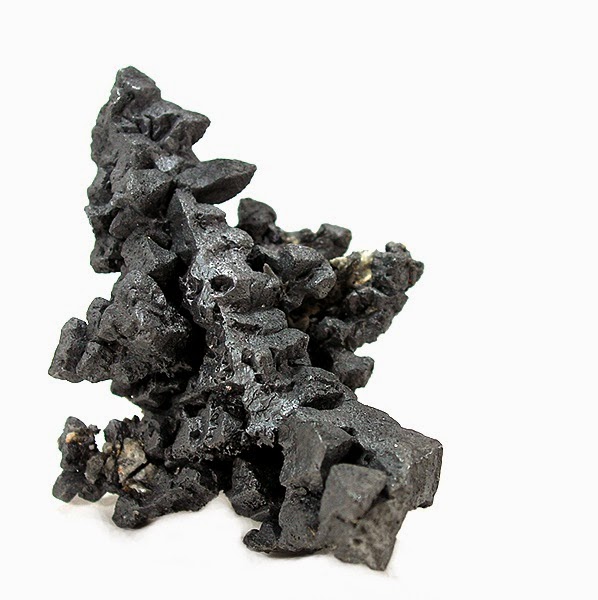
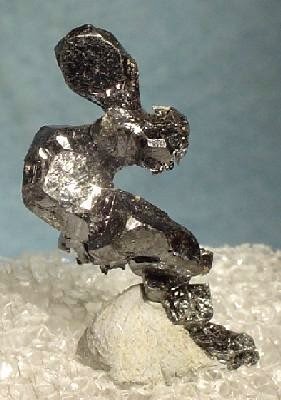
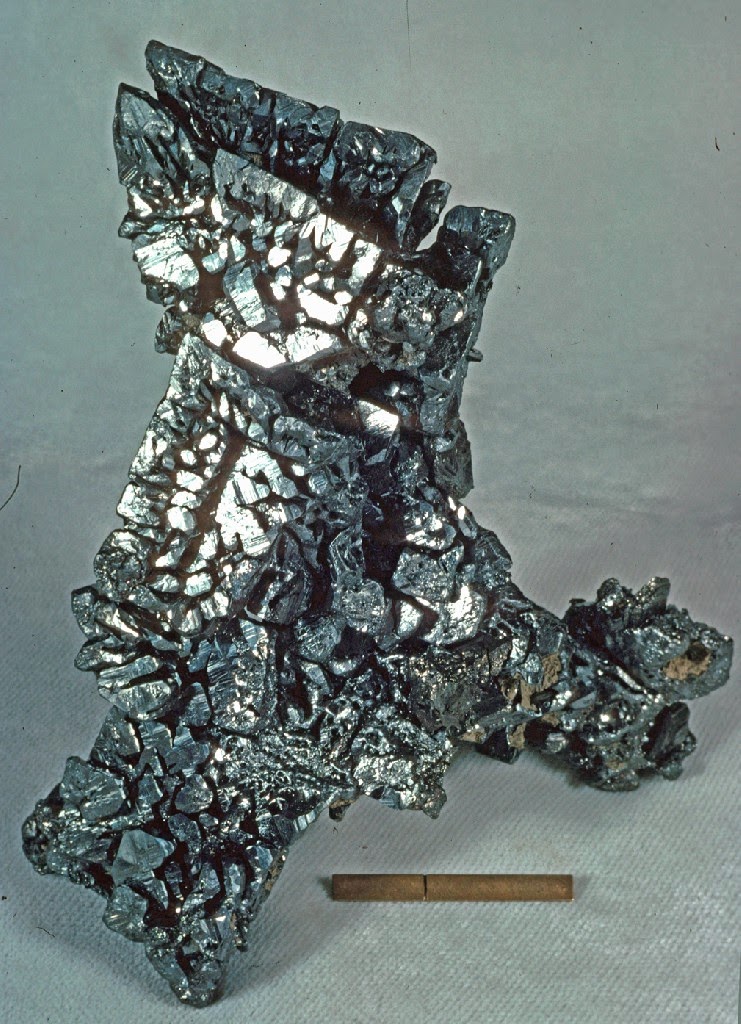
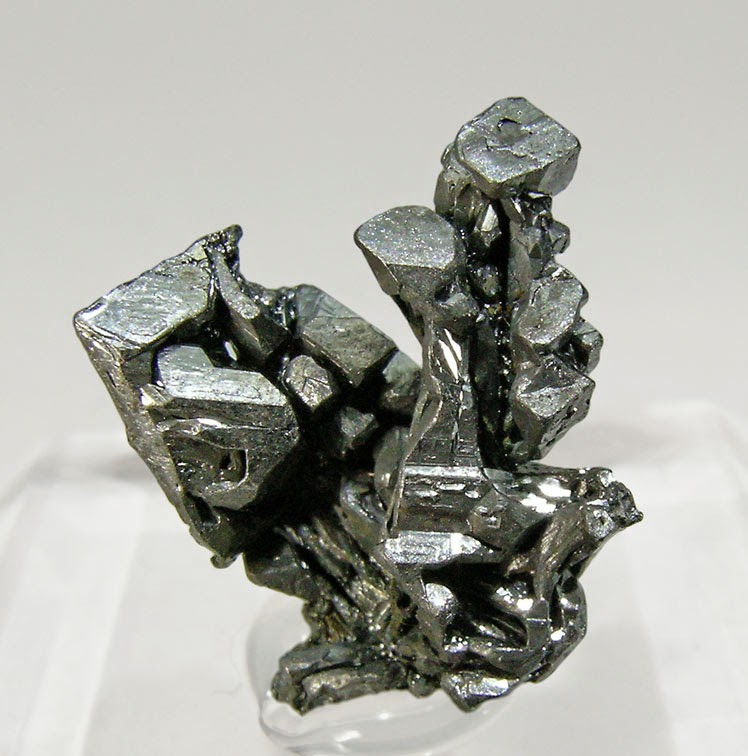
Lava Formations in Western U.S. Linked to Rip in Giant Slab of Earth
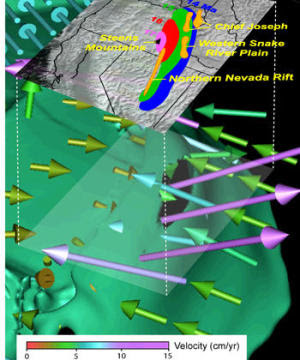
“When the slab is first opened there’s a little tear, but because of the high pressure underneath, the material is able to force its way through the hole. It’s like in the movies when a window breaks in an airplane that is at high altitude — since the cabin is at higher pressure, everything gets sucked out the window,” said Stegman, an assistant professor with Scripps’ Cecil H. and Ida M. Green Institute of Geophysics and Planetary Physics.
Liu and Stegman came upon their new mechanism by attempting to describe how the complicated structure of Earth’s mantle under the western U.S. developed during the past 40 million years. The final state of their model’s time-evolution matches the present day structure as imaged by the USArray, the National Science Foundation’s transportable seismic network of 400 sensor stations leapfrogging across the United States.
The John Miles Fellowship, the Cecil and Ida Green Foundation and the G. Unger Vetlesen Foundation funded the study.
Fukushima at Increased Earthquake Risk, Scientists Report
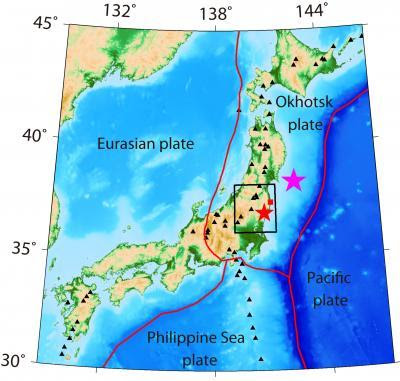