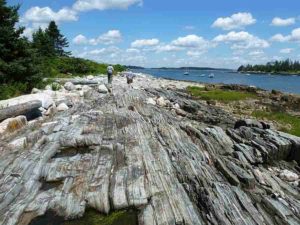
Earthquakes produce seismic waves with a range of frequencies, from the long, rolling motions that make skyscrapers sway, to the jerky, high-frequency vibrations that cause tremendous damage to houses and other smaller structures. A pair of Brown University geophysicists has a new explanation for how those high-frequency vibrations may be produced.
In a paper published in Geophysical Research Letters, Brown faculty members Victor Tsai and Greg Hirth propose that rocks colliding inside a fault zone as an earthquake happens are the main generators of high-frequency vibrations. That’s a very different explanation than the traditional one, the researchers say, and it could help explain puzzling seismic patterns made by some earthquakes. It could also help scientists predict which faults are likely to produce the more damaging quakes.
“The way we normally think of earthquakes is that stress builds up on a fault until it eventually fails, the two sides slip against each other, and that slip alone is what causes all the ground motions we observe,” said Tsai, an associate professor in Brown’s Department of Earth, Environmental and Planetary Sciences. “The idea of this paper is to evaluate whether there’s something other than just slip. The basic question is: If you have objects colliding inside the fault zone as it slips, what physics could result from that?”
Drawing from mathematical models that describe the collisions of rocks during landslides and other debris flows, Tsai and Hirth developed a model that predicts the potential effects of rock collisions in fault zones. The model suggested the collisions could indeed be the principal driver of high-frequency vibrations. And combining the collision model with more traditional frictional slip models offers reasonable explanations for earthquake observations that don’t quite fit the traditional model alone, the researchers say.
For example, the combined model helps explain repeating earthquakes — quakes that happen at the same place in a fault and have nearly identical seismic wave forms. The odd thing about these quakes is that they often have very different magnitudes, yet still produce ground motions that are nearly identical. That’s difficult to explain by slip alone, but makes more sense with the collision model added, the researchers say.
“If you have two earthquakes in the same fault zone, it’s the same rocks that are banging together — or at least rocks of basically the same size,” Tsai said. “So if collisions are producing these high-frequency vibrations, it’s not surprising that you’d get the same ground motions at those frequencies regardless of the amount of slip that occurs.”
The collision model also may help explain why quakes at more mature fault zones — ones that have had lots of quakes over a long period of time — tend to produce less damage compared to quakes of the same magnitude at more immature faults. Over time, repeated quakes tend to grind down the rocks in a fault, making the faults smoother. The collision model predicts that smoother faults with less jagged rocks colliding would produce weaker high-frequency vibrations.
Tsai says that more work needs to be done to fully validate the model, but this initial work suggests the idea is promising. If the model does indeed prove valid, it could be helpful in classifying which faults are likely to produce more or less damaging quakes.
“People have made some observations that particular types of faults seem to generate more or less high-frequency motion than others, but it has not been clear why faults fall into one category or the other,” he said. “What we’re providing is a potential framework for understanding that, and we could potentially generalize this to all faults around the world. Smoother faults with rounded internal structures may generally produce less high-frequency motions, while rougher faults would tend to produce more.”
The research also suggests that some long-held ideas about how earthquakes work might need revising.
“In some sense it might mean that we know less about certain aspects of earthquakes than we thought,” Tsai said. “If fault slip isn’t the whole story, then we need a better understanding of fault zone structure.”
Reference:
Victor C. Tsai, Greg Hirth. Elastic Impact Consequences for High‐Frequency Earthquake Ground Motion. Geophysical Research Letters, 2020; DOI: 10.1029/2019GL086302
Note: The above post is reprinted from materials provided by Brown University.