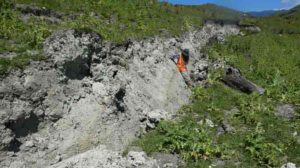
Curved scratches in rock faces may give clues to where big quakes could strike next, a study led by Victoria University of Wellington Master’s student Jesse Kearse has shown.
These scratches—or ‘slickenlines’—have been observed on fault lines for decades. Through his Master’s research—published last month in the journal Geology—Jesse was able to link the direction of the slickenlines with the direction a fault line ruptures during an earthquake, providing a record of how past earthquakes have moved on New Zealand’s fault lines and hints as to where future earthquake damage could occur.
Jesse’s research began when he was analysing the Kekerengu fault line as part of the Kaikōura Earthquake Surface Rupture Response Team immediately following the Kaikōura earthquake.
“We were mapping the ground ruptures that occurred around the Kekerengu fault as a result of the Kaikōura earthquake, and we found these intriguing curved marks,” Jesse says. “We wanted to uncover the process behind their formation, because we knew this was a field of research that wasn’t well understood.”
Alongside his supervisors Professor Tim Little of Victoria University of Wellington and Russ van Dissen from GNS Science, Jesse spent many weeks in the field, walking the ground ruptures from end to end—a total distance of 30 kilometres—documenting the ground deformation, and recording the slickenlines.
“After completing these observations, we took our data back to the lab for analysis,” Jesse says. “We knew that the rupturing of the Kekerengu fault had caused the ground to shift sideways by up to 12 m during the Kaikoura earthquake. Our analysis of the slickenlines not only confirmed this movement, but also provided further detailed information about how the fault moved—not in a straight line, but along a complicated, curved route.”
With help from seismologist Yoshi Kaneko from GNS, who uses computer programs to model the dynamics of earthquake ruptures, they were able to confirm that curved slickenlines like the ones they observed on the Kekerengu fault are related to the direction an earthquake moves along the fault.
It has long been known that the direction a fault ruptures can strongly affects the distribution of ground shaking and damage resulting from an earthquake, Jesse says.
“In the Kaikōura earthquake, for example, Wellington experienced much stronger shaking than Christchurch, even though the epicentre of the quake was much closer to Christchurch,” Jesse says. “This is because the Kekerengu fault ruptured towards the north, and so the earthquake energy was focused in that direction.”
Now Jesse and his colleagues can analyse the slickenlines in other faults to see how they have ruptured in the past, including prehistoric earthquakes that took place thousands of years ago.
“We might be able to better predict how they will rupture in the future and where the ground shaking and damage from earthquakes on these faults will occur,” Jesse says. “This will help plan for future quakes, including designing more resilient buildings and architecture in areas that could suffer more quake damage.”
Jesse hopes to see this research tested further across the world to confirm their findings and provide a new way for scientists to discover information about earthquakes.
“This research is very new, so while it’s very exciting it still needs to be tested and verified by the global earth science community,” Jesse says. “Hopefully this analysis will prove that these findings are of real benefit to the lives of people living in earthquake prone regions around the world.”
Reference:
Jesse Kearse et al. Curved slickenlines preserve direction of rupture propagation, Geology (2019). DOI: 10.1130/G46563.1
Note: The above post is reprinted from materials provided by Victoria University of Wellington.