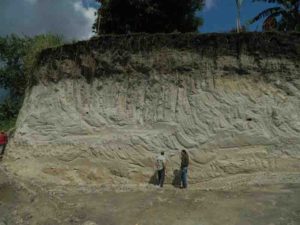
The Ilopango’s volcano eruption (also known as Tierra Blanca Joven or TBJ) occurred approximately 1,500 years ago. Pyroclastics currents were dispersed over much of the present territory of El Salvador and a volcanic ash column reached a height of 49 km, according to a new research published recently in Journal of Volcanology and Geothermal Research.
Dario Pedrazzi, researcher at the Institute of Earth Sciences Jaume Almera of the CSIC (ICTJA-CSIC) is the lead author of a research that, by means of analysing the TBJ ash (tephra) deposits, has reconstructed the eruptive process of what is thought to be the largest explosive eruption occurred in Central America in the Holocene (last 10,000 years).
“The TBJ eruption was initially studied several years ago, but such a complete stratigraphic study hadn’t yet been carried out and the physical parameters were not defined. The volcanic products dispersion was neither determined”, said Dario Pedrazzi.
This new study presents a complete stratigraphic description and the extent of the pyroclastic deposits of TBJ eruption, which are still present all over El Salvador and in some neighbouring countries. The study also describes the physical parameters of the different phases of the eruption that generated the surveyed deposits.
This research was carried out with the collaboration of researchers from the Centro de Geociencias of the Universidad Autónoma de México (UNAM) and the División de Geociencias aplicadas del IPICYT, Mexico; the Istituto Nazionale di Geofisica e Vulcanologia (INGV), Italy; the Oxford University, UK; the Oregon State University, USA and the Ministerio de Medio Ambiente y Recursos Naturales de El Salvador (MARN).
The authors of the study conducted a detailed field mapping of an area of about 200.000 km2 during three field campaigns in order to reconstruct the stratigraphy of the TBJ deposits and the relationship with other eruptive deposits.
“What called our attention was the thickness of the pyroclastic deposits. Some of them were up to 70m thick and reached distances of at least 40-50 km from the vent. We shouldn’t forget that San Salvador City and its metropolitan area were built over the pyroclastic deposits originated during TBJ eruption”, explains Dario Pedrazzi.
Researchers measured 82 stratigraphic sections all over El Salvador Country, but they finally focused on 21 outcrops. They collected nearly 200 samples from all the outcrops that were analysed afterwards in the MARN and UNAM laboratories to obtain the parameters needed to develop numerical simulations.
With all this data available, the authors of the study were able to reconstruct the TBJ eruption dynamics. They could identify a total of 8 units in the deposits that correspond to the different phases of the eruption.
“It was an eruption that started with pyroclastic surges in a very specific area. Then, there was a shift in the eruptive dynamics, characterized by ash fallout, and then it shifted again to another phase driven mainly by pyroclastic flows”, said Pedrazzi.
According to the researcher, “the eruption reached its climax with a series of pyroclastic flows probably linked to a caldera collapse. In the last phase, all the materials ejected previously were deposited by fallout mechanisms”. Some of these materials were transported and spread by the dominating winds and they reached distances as large as 100 km from the vent, especially fine grain ashes.
Thanks to the numerical simulations conducted, the authors of the study were able to estimate that during the final phase of the eruption, the column of volcanic ashes and gases (co-ignimbrite plume) reached a height of 49 km. Moreover, they calculated that the total bulk volume of ejected material was about 60 km3 of magma (30 km3 dense rock equivalent, which is the original volume of erupted magma), corresponding to a 6.8 magnitude eruption.
The study notes that the” Mayan populations living in the region would have been considerably affected “ and that the communities living in the territory within 50 km from the Ilopango Caldera were the ones who suffered a more direct impact. However, indirect effects on the social, economic and political systems derived from the eruption “probably affected a much wider area of Central America”.
According to the researchers, this study “represents the first and necessary step towards improved volcanic hazard assessment for the region” to mitigate volcanic risk for the large number of communities living around Ilopango Caldera, an active volcano whose last eruption was in 1879. It was then when some domes (Islas Quemadas) were formed inside the caldera. About 3 million people live currently within 30 kilometres of the caldera.
“The previous eruption occurred 8,000 years before TBJ eruption. The returning time of the last eruptions are shorter if we compare them with the first ones, occurred about 1.5 million years ago, although the volume of material ejected during the most recent eruptions is smaller”, said Dario Pedrazzi.
The Ilopango Caldera is located less than 10 km from San Salvador City, the capital of El Salvador, and it forms part of Volcanic Arc of El Salvador which includes a total of 21 active volcanoes, being one of the most active segments of the Central America Volcanic Arc.
This work is part of a project founded by CONACYT and lead by Dr.Gerardo Aguírre Díaz, UNAM researcher. The project is focused in the study of the Ilopango Caldera and its goal is to determine the potential hazard of volcanic supereruptions in Central America.
Reference:
Pedrazzi, D., Sunye-Puchol, I., Aguirre-Díaz, G., Costa, A., Smith, V., Poret, M., Dávila-Harris, P., Miggins,D., Hernández, W., Gutiérrez, E. (2019) “The Ilopango Tierra Blanca Joven (TBJ) eruption, El Salvador: Volcano-Stratigraphy and physical characterization of the major Holocene event of Central America”. Journal of Volcanology and Geothermal Research, 377, 81-102. DOI: 10.1016/j.jvolgeores.2019.03.0060377-0273
Note: The above post is reprinted from materials provided by ICTJA-CSIC. The original article was written by Jordi Cortés.