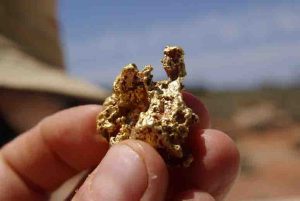
Gold Mines In The United States
Since the discovery of gold at the Reed farm in North Carolina in 1799, gold mining in the United States has continued. The first documented occurrence of gold occurred in Virginia in 1782. Some minor gold production took place in North Carolina as early as 1793, but did not create excitement.
The discovery on the Reed farm in 1799 which was identified as gold in 1802 and subsequently mined marked the first commercial production.
Gold production on a large scale began in 1848 with the California Gold Rush.
In the autumn of 1942, the War Production Board Limitation Order No. 208’s closure of gold mines during World War II was a major impact on production until the end of the war.
Alabama
Around 1830 in Alabama, gold was discovered shortly after the Georgia Gold Rush. The main districts were Cleburne County’s Arbacochee district, mostly from placer deposits, and Tallapoosa County’s Hog Mountain district, which produced 24,000 troy ounces (750 kg) of schist veins.
Alaska
In 1848, Russian explorers discovered gold placer in the Kenai River, but gold was not produced. Gold mining began from placers southeast of Juneau in 1870.[7] From 1880 to the end of 2007, Alaska produced a total of 40,300,000 troy ounces (1,250,000 kg) of gold. In 2015, 873,984 troy ounces (27,183.9 kg) of gold were produced by Alaskan mines, 12.7 percent of US production.
Fort Knox mine, a large open pit and cyanide leaching operation in the mining district of Fairbanks, is the largest gold producer. Fort Knox produced gold in 2015 at 401,553 troy ounces (12,489.7 kg). The gold mines of Pogo (283,000 ounces) and Kensington (128,865 ounces) and the polymetallic mine of Greens Creek (60,566 ounces) accounted for the rest of the gold production in 2015.
Arizona
More than 16 million troy ounces (498 tons) of gold were produced by Arizona. It is reported that gold mining in Arizona began in 1774 when Spanish priest Manuel Lopez ordered the Indians of Papago to wash gravel gold on the flanks of the Quijotoa Mountains, Pima County.
Gold mining continued until 1849, when the California Gold Rush lured the Mexican miners away. Other gold mining under Spanish and Mexican rule was carried out in the Santa Cruz County district of Oro Blanco and the Pima County district of Arivaca.
California
Spanish prospectors found gold about ten miles north-east of Yuma, Arizona, in the Potholes district between 1775 and 1780, along the Colorado River, in present-day Imperial County, California. The gold from dry placers has been recovered. Other placer deposits were quickly found on the west bank of the Colorado River, including the districts of Picacho and Cargo Muchacho.
Placer gold deposits were found in 1828 in San Ysidro County, 1835 and 1842 in Los Angeles County, San Francisquito Canyon and Placerita Canyon.
California’s gold production peaked at 3.9 million troy ounces (121 tonnes) that year in 1852. But in the early years, the placer deposits worked were quickly exhausted, and production collapsed. Hardrock mining (called quartz mining in California) began in 1849 and hydraulic mining of placer started in 1852.
Colorado
During the Peak Gold Rush in the vicinity of present-day Denver in 1858, gold was discovered, but the deposits were small. In January 1859, the first major gold discoveries in Colorado were in the district of Central City-Idaho Springs.
Only one Colorado mine is still producing gold, the Cripple Creek & Victor Gold Mine in Victor near Colorado Springs, a Newmont Mining Corporation-owned open-pit heap leach operation that produced 360,000 troy ounces (11,000 kg) of gold in 2018.
Florida
During the late 19th century, at the site where Mike Roess Gold Head State Park is today, small amounts of gold were mined commercially in North Eastern Florida. There are no records of the amount of gold produced, but the finding was insufficient to keep the operation running commercially, and within a matter of months the small amount of pay dirt has been depleted.
Georgia
Georgia has a total historical production of gold from 1830 to 1959 of 871,000 troy ounces (27,100 kg). Although the state is not a gold producer at the moment, historically important.
Idaho
In 1860, at the juncture where Canal Creek meets Orofino Creek, Gold was first discovered in Idaho, in Pierce.
The leading historic gold-producing district is Boise Basin in Boise County, discovered in 1862, producing 2,9 million troy ounces (90,2 tonnes), mostly from placers.
The French district of Idaho County Creek-Florence began in the 1860s, producing about 1 million troy ounces (31 tonnes) from placers. The district of Silver City in Owyhee County started producing in 1863 and produced over 1 million troy ounces (31 tons), mostly from lode deposits.
The district of Coeur d’Alene in Shoshone County produced 44,000 troy ounces (1,400 kg) of gold as a by-product of silver mining.
The Silver Strand mine and the Bond mine were active gold mines in Idaho in 2006.
Maryland
Gold was reported as early as 1830 in Maryland, but the result was no production. Placer gold was discovered by California Union soldiers at Great Falls near Washington, DC in 1861 during the American Civil War. A number of mines were opened in Montgomery County on gold-bearing quartz veins after the war. Since 1951, there has been no gold production reported. There were about 6,000 troy ounces (190 kg) of total production.
Michigan
From the Ropes gold mine northeast of Ishpeming in Marquette County, Michigan, about 29,000 troy ounces (900 kg) of gold were produced. Originally operating from 1880 to 1897 and reopened from 1983–1989, the underground mine extracted gold in peridotite from quartz veins.
Montana
Gold was first discovered in 1852 in Montana, but mining did not start until 1862, when gold placers were found in 1862 in Bannack, Montana. The resulting gold rush resulted in more placer discoveries, including in 1863 in Virginia City, and in 1864 in Helena and Butte.[28 ] The Atlantic Cable Quartz Lode was located in 1867.
The Montana Tunnels mine and the Golden Sunlight mine are currently active hardrock gold mines. The Browns Gulch placer and the Confederate Gulch placer are active gold placers. The Stillwater igneous complex also produces gold from three platinum mines: the Stillwater mine, the Lodestar mine, and the East Boulder Project.
Nevada
Nevada is the nation’s leading gold producing state, producing 5,467,646 troy ounces (170,06 tons) in 2016, accounting for 81% of US gold and 5.5% of world production. Much of Nevada’s gold comes from large open pit mining and recovery from heap leaching.
Some of the major mining companies in the world, including Newmont Mining, Barrick Gold, and Kinross Gold, operate state-owned gold mines. Cortez, Twin Creeks, Betz-Post, Meikle, Marigold, Round Mountain, Jerritt Canyon and Getchell are active major mines.
New Mexico
Gold was first discovered in the “Old Placers” district of the Ortiz Mountains, Santa Fe County, New Mexico, in New Mexico in 1828. Following the discovery of placer gold, a nearby lode deposit was discovered.
Two prospectors collected float in 1877 near Hillsboro, New Mexico in the area of the future Opportunity Mine, which was tested at $160 per ton in gold and silver. In the nearby Rattlesnake vein, ore was soon discovered and a placer deposit of gold was found in the Rattlesnake and Wicks gulches in November. Before 1904, total production was about $6,750,000.
All gold production in New Mexico in 2007 (13,000 troy ounces (400 kg)) came from two large open pit mines in Grant County as a by-product of copper mining. Two primary gold mines are being prepared for production, however: the Rio Arriba County Northstar mine and the San Lorenzo Claims mine in Socorro County.
North Carolina
After the discovery of a 17-pound (7.7 kg) gold nugget by 12-year-old Conrad Reed in a stream at his father’s farm in 1799, North Carolina was the site of the first gold rush in the United States. The Reed Gold Mine in Cabarrus County, North Carolina, southwest of Georgeville, produced about 50,000 troy ounces (1,600 kg) of gold from deposits of lode and placer.
Gold was produced in 15 districts, nearly all of them in the state’s Piedmont region. The total production of gold is estimated at 1.2 million ounces of troy (37.3 tonnes).
Oregon
Although gold mines are spread across much of Oregon, nearly all the gold produced comes from two main areas: the Klamath Mountains in southwestern Oregon, including Coos, Curry, Douglas, Jackson and Josephine counties ; and the Blue Mountains in northeastern Oregon, mostly in Baker and Grant counties.
Illinois prospectors discovered placer gold in southwest Oregon’s Klamath Mountains in 1850, beginning a rush to the area. Deposits of Lode gold have also been discovered. Travelers bound for the Willamette Valley along the Oregon Trail are said to have discovered gold in northeastern Oregon in 1845, but earnest mining did not begin until 1861.
Pennsylvania
Approximately 37,000 troy ounces (1,200 kg) of gold were produced five miles south of Lebanon, Lebanon County, Pennsylvania from the Cornwall Iron Mine. Although since 1742 the deposit produced iron, no gold from the mine was reported until 1878.
South Carolina
There were lode gold mines along the Carolina Slate Belt in South Carolina. The Haile deposit was discovered in Lancaster County in 1827, and between that time and 1942, at least 257,000 troy ounces (8,000 kg) of gold were intermittently extracted when the gold mine was ordered to be closed as non-essential to the war effort.
The deposit was mined for associated sericite at the beginning of 1951, which was used as a white filler. Gold is associated with silicon, kaolinite, and pyritic alteration of felsic metavolcanics of greenschist grade. The mine reopened in the 1980s as an open pit, operating until 1992.
OceanGold Corp. restarted mining at the Haile deposit 2016. The company expects to produce an average of 126,700 ounces of gold per year for 13.25 years.
From 1828 to 1995, the Brewer mine was operating and is now a federal Superfund site.
From 1988 to 1999, Kennecott Minerals operated the Ridgeway open-pit gold mine, and Kennecott is now reclaiming the land.
Between 1990 and 1994, the Barite Hill mine operated.
South Dakota
South Dakota’s only operating gold mine is the Wharf mine at Lead, a Coeur Mining open pit heap leach operation that produced 109,000 ounces of gold in 2016.
Tennessee
In 1827, on Coker Creek in Monroe County, Tennessee, Placer gold was discovered. Some 9,000 troy ounces (280 kg) were produced by the district. Approximately 15,000 troy ounces (470 kg) of gold were recovered from Ducktown, Tennessee’s massive sulfide copper ores.
Texas
Some prospects were excavated on the central Texas Llano Uplift for gold. Gold prospects include the Heath mine and the Babyhead district in both Llano County and Gillespie County’s Central Texas mine. There is no known production of gold, if any. Historically, Texas may have been home to the Lost Nigger Gold Mine.
Utah
Most gold produced today in Utah is a by-product of Salt Lake City’s huge Bingham Canyon copper mine. In 2013, 192,300 troy ounces (5,980 kg) of gold were produced by the Bingham Canyon mine. Bingham Canyon has produced over 23 million ounces (715 tons) of gold over its lifetime, making it one of the largest gold producers in the United States.
The Salt Lake County Barneys Canyon mine, the last primary gold mine operating in Utah, stopped mining in 2001, but is still recovering gold from its heap leaching pads. The production of Utah gold in 2006 was 460,000 troy ounces (14,000 kg).
Virginia
Most of Virginia’s gold mining was concentrated in the Virginia Gold-Pyrite belt in a line running north-east to south-west through Fairfax, Prince William, Stafford, Fauquier, Culpeper, Spotsylvania, Orange, Louisa, Fluvanna, Goochland, Cumberland, and Buckingham counties. There was also some gold mining in counties like Halifax, Floyd, and Patrick.
Washington
Gold was first discovered as a placer deposit in the Yakima Valley in Washington in 1853. State production never exceeded 50,000 troy ounces per year until the mid-1930s, when large hard rock deposits were built near the deposits of Chelan Lake and Wenatchee in Chelan County, and the Republic deposit in Ferry County. Production is estimated at 2,3 million ounces through 1965.
Wyoming
Gold was found in the present Fremont County in 1842 in the South Pass-Atlantic City-Sweetwater district. The placers were intermittently worked until 1867 when the first important gold vein was discovered and the area was rushed by prospectors and miners.
The miners were served by the cities of South Pass City, Atlantic City, and Miner’s Delight. By 1875, the district was almost deserted and only intermittently subsequently worked. The total production of gold was approximately 300,000 troy ounces (9,300 kg). The district became a major iron mine site in 1962.