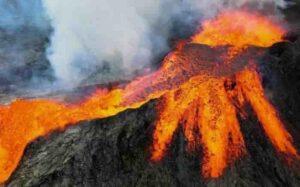
Scientists predict from geochemical data that Iceland is entering a new volcanic era that will last for decades, possibly centuries. Under an hour’s drive from the country’s capital city, the ongoing eruptions pose considerable risks for economic disruption, and they leave evacuated communities uncertain of a possible return.
Iceland’s ongoing volcanic eruptions may continue on and off for years to decades, threatening the country’s most densely populated region and vital infrastructure, researchers predict from local earthquake and geochemical data.
The eruptions on the Reykjanes Peninsula have forced authorities to declare a state of emergency, with a series of eight eruptions having occurred since 2021. This southwestern region is home to 70 percent of the country’s population, its only international airport, and several geothermal power plants that supply hot water and electricity. The most recent eruption in May through June triggered the evacuation of residents and visitors of the Blue Lagoon geothermal spa, a popular tourist attraction, for the third time in more than two months.
Although Iceland sees regular eruptions because it sits above a volcanic hot spot, the Reykjanes Peninsula has been dormant for 800 years. Its last volcanic era continued over centuries however, prompting scientists to predict the renewed volcanism to be the start of a long episode.
Under an hour’s drive from the island’s capital city Reykjavík, the eruptions pose considerable risks for economic disruption, and they leave evacuated communities uncertain of a possible return.
An international team of scientists has been watching the volcanoes over the past three years. Analyzing seismic tomography imaging and the composition of lava samples, they’ve uncovered parts of the geological processes behind the new volcanic era. They predict the region may have to prepare for recurring eruptions lasting years to decades and possibly centuries.
The researchers report their findings in a paper published June 26 in the journal Terra Nova. The project included collaborations from the University of Oregon, Uppsala University in Sweden, University of Iceland, Czech Academy of Sciences and University of California, San Diego. The work follows an earlier Nature Communications study of the initial Reykjanes eruptions in 2021.
Almost all of Iceland’s island is built from lava, said Ilya Bindeman, a volcanologist and earth sciences professor at the UO. The country sits on the Mid-Atlantic Ridge, the tectonic plate boundary that causes North America and Eurasia to push further apart. The drifting of these plates can spark volcanic eruptions when hot rock from the earth’s mantle — the middle and largest layer of the planet — melts and rises to the surface.
Although scientists know the origin of Reykjanes Peninsula’s current eruptions is plate movement, the kind of magma storage and plumbing systems that feed them are unidentified, Bindeman said. The peninsula consists of eight volcanically active sites, so understanding whether there is one shared magma source or multiple independent ones and their depth can help predict the duration and impact of these eruptions.
Using geochemical and seismic data, the researchers investigated whether the magma of the initial eruptions from one volcano in the peninsula from 2021 to 2023 came from the same source as the magma in the recent eruptions of a different volcano to the west.
Bindeman specializes in isotopic analysis, which can help identify the “fingerprint” of magma. Magma is made of mostly eight elements, including oxygen and hydrogen, and 50 different trace elements in smaller concentrations and various ratios. The unique combination of trace elements can help differentiate magma sources from one another. Scientists can also measure the abundance of isotopes, elements with the same chemical property but different masses, in the magma. There are three different isotopes of oxygen, for example, Bindeman said.
“In the air we breathe, there’s a mixture of these oxygen isotopes and we don’t feel the difference,” he said. “Their differences are usually not important for chemical reactions but are important to recognize as their relative abundances in magma can differentiate one magma source from another.”
Analyzing samples of lava rock from two different volcanoes in the peninsula, their similar fingerprints implied a shared magma storage zone below the peninsula. Imaging of earth’s interior based on local earthquakes also suggested the existence of a reservoir about 5.5 to 7.5 miles in the earth’s crust, the shallowest layer.
However, that storage is ultimately fed by the melting rock deeper in the mantle, which can cause eruptions that last decades, with hundreds of square miles of magma surfacing, Bindeman said. Iceland’s hotspot also will have no problem fountaining that flow, he said.
Although this marks the beginning of potentially persistent volcanic episodes in Iceland, the researchers can’t precisely predict yet how long the episodes and the gaps between each will last.
“Nature is never regular,” Bindeman said. “We don’t know how long and how frequently it will continue for the next ten or even hundred years. A pattern will emerge, but nature always has exceptions and irregularities.”
Discussions are continuing on plans to safely drill into the volcanic sites to glean insights into the geological processes driving the eruptions.
Because the volcanic activity is less volatile and explosive than eruptions in other countries, it provides a rare opportunity for scientists to approach fissures actively erupting lava, Bindeman said. He called it a “natural laboratory” both astonishing and chilling.
“When you witness a volcanic eruption, you can feel that these are the massive forces of nature, and you yourself are very small,” Bindeman said. “These events are ordinary from the geological scale, but from the human scale, they can be devastating.”
Reference:
Valentin R. Troll, Frances M. Deegan, Thor Thordarson, Ari Tryggvason, Lukáš Krmíček, William M. Moreland, Björn Lund, Ilya N. Bindeman, Ármann Höskuldsson, James M. D. Day. The Fagradalsfjall and Sundhnúkur Fires of 2021–2024: A single magma reservoir under the Reykjanes Peninsula, Iceland? Terra Nova, 2024; DOI: 10.1111/ter.12733
Note: The above post is reprinted from materials provided by University of Oregon. Original written by Leila Okahata.