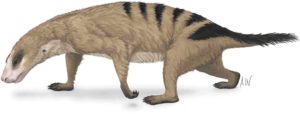
Bats fly, whales swim, gibbons swing from tree to tree, horses gallop, and humans swipe on their phones — the different habitats and lifestyles of mammals rely on our unique forelimbs. No other group of vertebrate animals has evolved so many different kinds of arms: in contrast, all birds have wings, and pretty much all lizards walk on all fours. Our forelimbs are a big part of what makes mammals special, and in a new study in the Proceedings of the National Academy of Sciences, scientists have discovered that our early relatives started evolving diverse forelimbs 270 million years ago — a good 30 million years before the earliest dinosaurs existed.
“Aside from fur, diverse forelimb shape is one of the most iconic characteristics of mammals,” says the paper’s lead author Jacqueline Lungmus, a research assistant at Chicago’s Field Museum and a doctoral candidate at the University of Chicago. “We were trying to understand where that comes from, if it’s a recent trait or if this has been something special about the group of animals that we belong to from the beginning.”
To determine the origins of mammals’ arms today, Lungmus and her co-author, Field Museum curator Ken Angielczyk, examined the fossils of mammals’ ancient relatives. About 312 million years ago, land-dwelling vertebrates split into two groups — the sauropsids, which went on to include dinosaurs, birds, crocodiles, and lizards, and the synapsids, the group that mammals are part of. A key difference between sauropsids and synapsids is the pattern of openings in the skull where jaw muscles attach. While the earliest synapsids, called pelycosaurs, were more closely related to humans than to dinosaurs, they looked like hulking reptiles. Angielczyk notes, “If you saw a pelycosaur walking down the street, you wouldn’t think it looked like a mammal — you’d say, ‘That’s a weird-looking crocodile.'”
About 270 million years ago, though, a more diverse (and sometimes furry) line of our family tree emerged: the therapsids. “Modern mammals are the only surviving therapsids — this is the group that we’re part of today,” explains Lungmus. Therapsids were the first members of our family to really branch out — instead of just croc-like pelycosaurs, the therapsids included lithe carnivores, burly-armed burrowers, and tree-dwelling plant-eaters.
Lungmus and Angielczyk set out to see if this explosion of diversity came with a corresponding explosion in different forelimb shapes. “This is the first study to quantify forelimb shape across a big sample of these animals,” says Lungmus. The team examined the upper arm bones of hundreds of fossil specimens representing 73 kinds of pelycosaurs and therapsids, taking measurements near where the bones joined the shoulder and the elbow. They then analyzed the shapes of the bones using a technique called geometric morphometrics.
When they compared the shapes of arm bones, the researchers found a lot more variation in the bones of the therapsids than the pelycosaurs. They also noted that the upper part of the arm, near the shoulder, was especially varied in therapsids — a feature that might have let them move more freely than the pelycosaurs, whose bulky and tightly-fitting shoulder bones likely gave them a more limited range of motion.
Lungmus and Angielczyk found that a wide variety of different forelimb shapes evolved within the therapsids 270 million years ago. “The therapsids are the first synapsids to increase the variability of their forelimbs — this study dramatically pushes that trait back in time,” says Lungmus. Prior to this study, the earliest that paleontologists had been able to definitively trace back mammals’ diverse forelimbs was 160 million years ago. With Lungmus and Angielczyk’s work, that’s been pushed back by more than a hundred million years.
The researchers note that the study helps explain how mammals evolved traits that have made us what we are today. “So much of what we do every day is related to the way our forelimbs evolved — even simple things like holding a phone,” says Angielczyk.
“This is something that’s so cool about our evolutionary lineage,” says Lungmus. “These animals are in the same group as us — part of what makes this research compelling is that these are our relatives.”
Reference:
Jacqueline K. Lungmus, Kenneth D. Angielczyk. Antiquity of forelimb ecomorphological diversity in the mammalian stem lineage (Synapsida). Proceedings of the National Academy of Sciences, 2019; 201802543 DOI: 10.1073/pnas.1802543116
Note: The above post is reprinted from materials provided by Field Museum.